Translate this page into:
The Influence of Body Mass Composition, Anthropometry, Body Posture and Head Supported Mass on Vibration Transmissibility: A Seated Position Explorative Study
Abstract
Background:
Occupational exposure to whole-body vibration (WBV) may have detrimental effect on health, advancing to low backache (LBA), early degeneration of the lumbar spine and disc herniation. The strong association of use of head supported mass (HSM), anthropometry, body posture and body mass composition with vibration transmission may help in detecting the hazard and imposing remedial measures. This study, analyses association between body mass, anthropometry, head supported mass and posture to WBV in a group of young Indian male volunteers.
Methods:
In this observational cohort study, 23 healthy, Indian males volunteered for participation. Seat to head, Z-axis vibration transmissibility (VTz) was recorded for frequency bandwidth of 4 -21 Hz for exposure duration of 30 min. The alteration in transmissibility was studied for HSM and body posture. Association with anthropometric factors (Age, sitting height, waist and hip size) and body mass composition (Fat%, water%, DLW, Bio-IMD and BMI) characteristics of participants were also studied. Information on musculoskeletal pain status was collected by questionnaire feedback. The influence of experiment variables and body characteristics on transmission were analysed by ANOVA, correlation and regression statistical methods.
Results:
The VTz transmisibility in seated posture was maximum at 4 Hz followed by 6 and 8 Hz. The repeated measure ANOVA demonstrated that vibration transmissibility changed with frequency (95% CI, F=170.83, p<0.01) and posture (95% CI, F=7.51, p=0.011). The HSM effect was insignificant but its interaction effect with other experimental factors were influential on transmissibility. Average VTz were positively correlaed to waist (r = 0.43, r = 0.54) and hip size (r = 0.43) and to sitting height 4Hz 8Hz 6Hz (r6Hz= 0.52, r18Hz= 0.46) at 4, 6 , 8 and 18 Hz. Simple regression analysis gave significant β for these factors. However, multiple 6Hz 18Hz regression could not associate transmisibility with anthropometric factors.
Conclusion:
Transmission of vibration to the body is a complicated phenomenon because of nonlinearities in the human musculoskeletal system. Based on the experimental and statistical analytical investigation, we could prove that there was no significant correlation of body mass composition to vibration transmission. It can also be concluded that anthropometric parameters had strong association with vibration transmission only at specific frequencies.
Keywords
Whole-body vibration
Vibration transmissibility
Body composition
Anthropometry
Body posture
correlation
simple and multiple regression
Introduction
Whole body vibration (WBV) exposure of long duration and high magnitude increases the risk of spinal disorder. High prevalence of vibration induced low back pain, neck pain, even shoulder and thoracic pain are inherent in rotary wing aviation. Although the effect may vary based on anthropometric and body mass characteristics of the individual, the vibration transmissibility depends on the external factors like vibration characteristics, duration of exposure, seat dynamics, posture, head supported devices and restraint systems.
It also depends on the internal factors like age, anthropometry (height, weight, waist and hip size) body composition (lean mass, BMI, fat and water %) [1]. Research studies aimed at exploration of vibration transmission, support its potential in bone training. Substantial amplification of peak acceleration can be seen between 10 and 40 Hz for the ankle, 10 and 25 Hz for the knee, 10 and 20 Hz for the hip and at 10 Hz for the spine [2].
HSM combined with vibration exposure, potentially degrades aircrew performance and safety. The changes can be correlated to head orientation, body posture and centre of gravity (CG) shift of the helmet. Helmet and NVG mass increases stress on neck and trapezius muscle activity. However, balance of mass by addition of counterweight, minimizes metabolic and hemodynamic stress [3, 4]. The gender and anthropometric influences are observed on biodynamic response to vibration exposure. The excitation magnitude was dependent on body mass, BMI and hip circumference in males and females [5]. Whole body vibration combined with poor posture increases the risk of low backache in pilots, primarily in rotary wing aircraft. The response of the erector spine muscles to vibration can be studied using electromyogram (EMG) activity [6]. Degenerative changes related to age and vibration stress has been observed in rotary wing pilots.
Researchers are making efforts to find out the predictors of aviation related low back pain. Questionnaire based information on effect of age, anthropometry, flying hours, smoking habits, lifestyle on back pain were collected. Oswestry score indicated that long flying hours, sedentary lifestyle, impaired back muscle endurance and age, negatively affect low back disability status [7].
With the above background, primary aim of this study was to measure seat to head vibration transmissibility in male volunteers. Thereafter, compare it to extrinsic and intrinsic body parameters as co-variates to associate them with exposure outcomes.
Material and Methods
Study Design
This repeated measure observational cohort study was conducted on 23 male volunteers to investigate the relationship between body composition, anthropometry and sitting posture to vibration transmissibility. The block diagram of the study design is given in Fig 1. This study was part of larger project designed to investigate back strength, vibration transmission and their role in LBA.
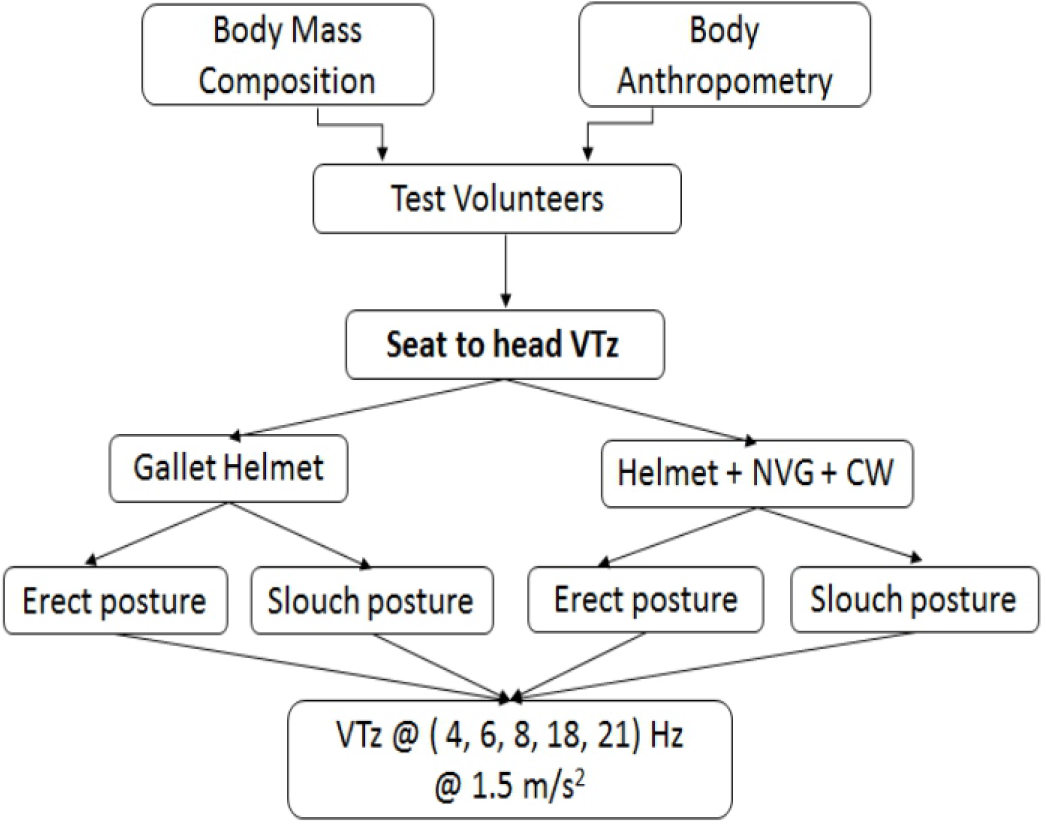
- Study design
Inclusion /Exclusion Criteria
The volunteers who participated in the study were free from any apparent contraindication to vibration exposure (e.g. Cases with osteoporosis). The participants were in good health, with no history of significant illness or injury to spine or limbs. On arrival at the experimental site, participants were explained about the test protocol and duration of the test. The subjects were instructed not to take the support of the seat back-rest. The hands were allowed to be kept on the arm rest and the feet were firmly placed on vibration platform.
Laboratory Experimental Setup
The experiment involved 4 sequences of data measurements. In set 1 and 2, intrinsic and extrinsic body parameters were measured. The body composition were measured non-invasively using 2-channel Bio-impedance analyzer (Bodystat 1500). The subjects' anththropometric data was measured manually on the indegenously developed IAM anthropometry platform. Thereafter, uniaxial vibration was administered using a computerized, vibration simulator, that has been indegenously developed at IAM (Fig. 2). The Z-axis oscillation capability of the simulator is 1-35 Hz and 1-20 m/s and has a magazine weight capacity of 180 kg. Uniaxial, analogue accelerometer measured vibration transmissibility as head acceleration.
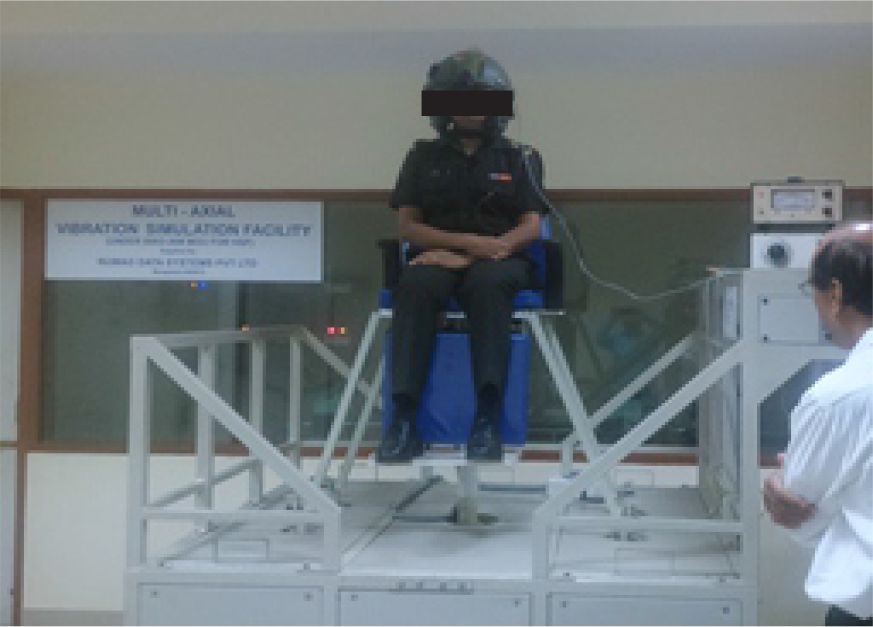
- Vibration simulator lab at IAM, IAF
Intrinsic and Extrinsic Body Parameters
The Participants’ anthropometric parameters (height, sitting height, weight, hip and waist size) were measured in anthropometry lab of the Human Engineering Dept (Fig-3). The body composition parameters (Body Fat, lean mass, dry lean weight (DLW), body water, BMR and BMI) were measured using Bio-electrical Impedance Analysis (BIA) method by placing electrodes colinearly as depicted in Fig 4.
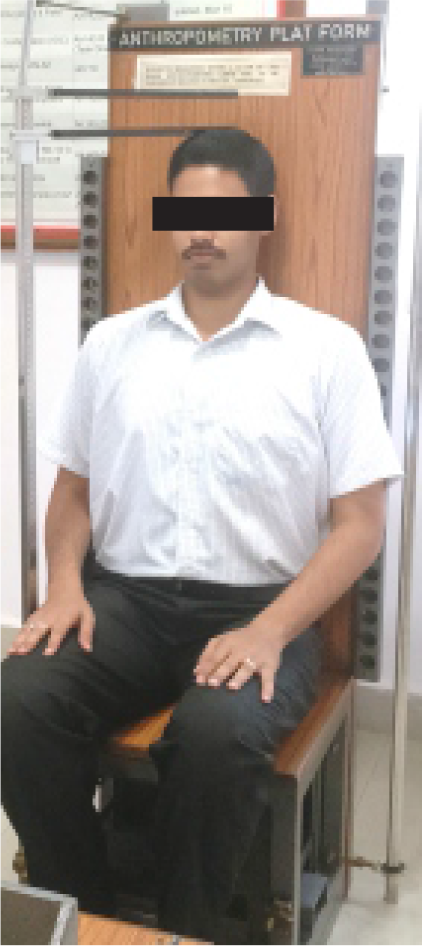
- Anthropometric measurements on IAM Anthropometric platform
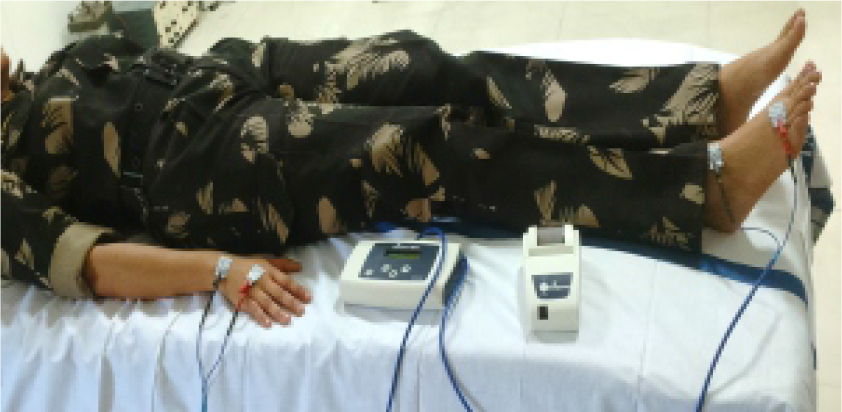
- Sitting height measurement using anthropometry platform and body mass composition measurement using bodystat- 1500
WBV Exposure and Transmissibility
The test participants were exposed to mechanical Z- axis vibration on electro-hydrolically oscillated chair (Fig 2) in seated posture. The sinusoidal low frequency bandwidth was 4 - 21 Hz at 1.5 m/s for 30 min. This stimulus was within the limits of ISO-2631 standard. Real time, seat to head vibration transmissibility in Z-axis (VTz) was measured by mounting accelerometer on the helmet, donned by the volunteers. Transmissibility was ratio of set acceleration to the acceleration measured at the level of the head. It was ensured that the subjects' helmet chin-strap was optimally adjusted. The transmisibility was monitored in straight and slouch posture and test was repeated with NVG–CW assembly.
Data Analysis
Analysis was carried out for vertical Z-axis vibration. A 2 x 2 x 5 repeated measure ANOVA (α set at 0.05), Pearson's correlation, univariate and multivariate regression probed potential influence of body composition, anthropometry, posture and head worn mass on vibration transmissibility.
Results
Participant Information
The participants had a mean age of 20.17±6.64 years, mean weight of 69.91±9.23 kg and mean sitting height of 90.93±2.55 cm. The mean values of Fat%, DLW, Water, BMI and Bio-IMD were 6.38±5.06%, 18.39±3.26 kg, 45.95±4.67%, 22.74±1.97 kg/m and 427.83±47.02 Ω respectively. The values are presented in Table 1 and body mass composition is graphically presented in Fig 5.
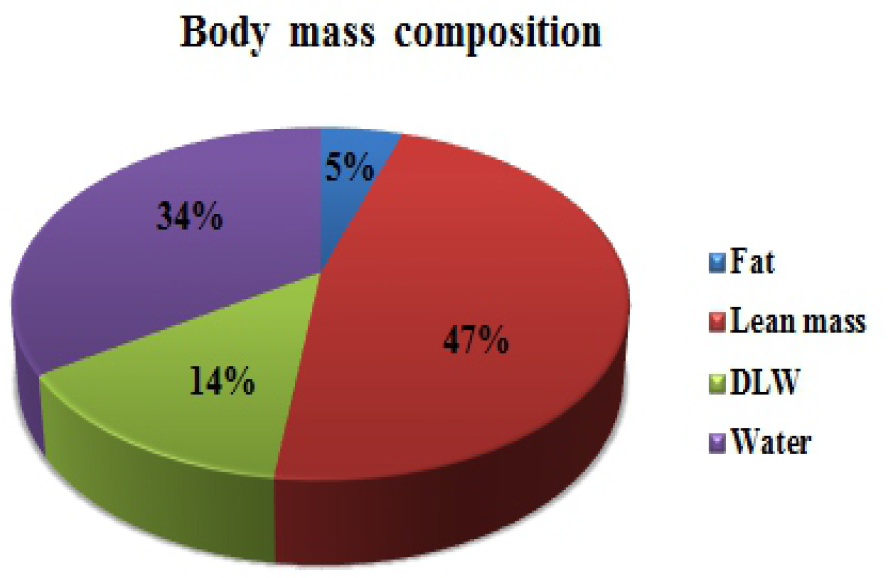
- Body mass composition, fat, lean mass, DLW and water in male volunteers
Items | Age (years) | Height (cm) | Weight (kg) | Waist (cm) | Hip (cm) | Sitting height (cm) |
---|---|---|---|---|---|---|
Mean± SD | 20.17±6.64 | 175.09±6.87 | 69.91±9.23 | 90.57±5.95 | 99.00±6.24 | 90.93±2.55 |
Fat% | Lean mass% | DLW (kg) | Water % | BMI (kg/m2) | Bio- IMD (Ω) | |
Mean± SD | 6.38±5.06 | 63.13±10.08 | 18.39±3.26 | 45.95±4.67 | 22.74±1.97 | 427.83±47.02 |
Vibration Transmissibility
None of the participants felt any discomfort or numbness during the test run. The average transmissibility was maximum at 4 Hz (Table 2). The next highest transmission was recorded at 8 Hz. Difference in transmissibility was observed for head supported mass and posture. The transmissibility data in graphical format is presented in Fig 6.
HSM | Sitting Posture | VTz (m/s2) | ||||
---|---|---|---|---|---|---|
4 Hz | 6 Hz | 8 Hz | 18 Hz | 21 Hz | ||
Gallet Helmet | Erect | 1.46 | 0.54 | 0.74 | 0.45 | 0.41 |
Slouch | 1.45 | 0.61 | 0.76 | 0.42 | 0.29 | |
Helmet + NVG + CW | Erect | 1.64 | 0.63 | 0.87 | 0.41 | 0.32 |
Slouch | 1.56 | 0.60 | 0.82 | 0.34 | 0.25 |
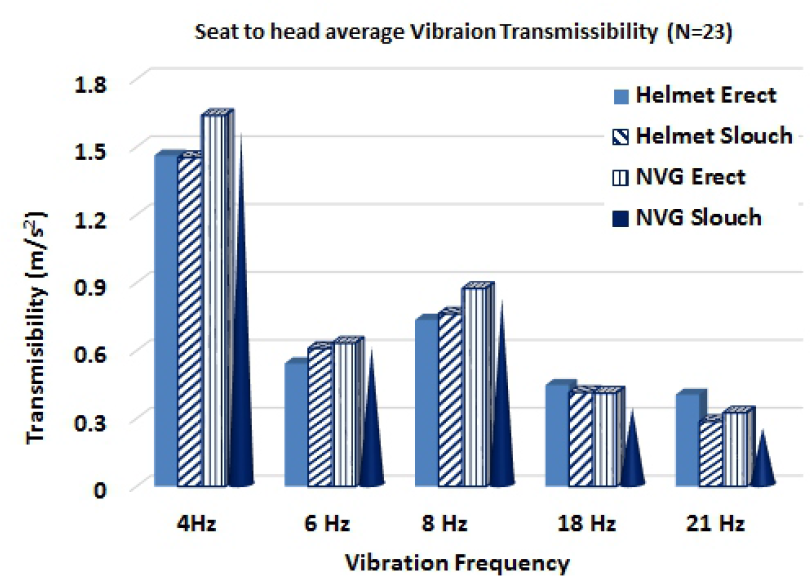
- Seat to head Vibration Transmissibility for frequency sweep of 4 – 21 Hz
Transmissibility Relationships
The results obtained through repeated measure ANOVA, considering three main factors (HSM, Posture, Frequency) suggested that the mean transmissibility was significant for posture (95% CI, F=7.51, p=0.011) and frequency (95% CI, F=170.83, p <0.01). The statistical significance for HSM and VTz was 95% CI, F=3.80, p=0.064. The results also indicated significant interaction of the main factors (Table 3). The Eta-squared effect size(η =0.984), indicated that more than 90% of the variance in vibration transmissibility was accounted for by the frequency. Bonferroni post-hoc analysis revealed that significant transmission took place at 4, 6 and 8Hz.
Test conditions | ANOVA VTz significance | Post hoc significance | |
---|---|---|---|
F- ratio | p - level | p -level | |
HSM | 3.80 | 0.064 | - |
PST | 7.51 | 0.011 | 0.011 |
FRQ | 170.83 | 0.000 | 4 Hz ( p6Hz< 0.000, p8Hz< 0.000, p18Hz< 0.000,p21Hz< 0.000) |
HSM*PST | 5.23 | 0.032 | NVG_Erect (pHelmet_Erect=0.007,pHelmet_Slouch <0.001, pNVG_Slouch =0.002) |
HSM*FREQ | 10.81 | 0.000 | - |
PST *FREQ | 2.68 | 0.036 | - |
HSM*PST * FREQ | 2.75 | 0.032 | - |
HSM - Head Supported Mass, PST -erect and slouch posture, FREQ - Vibration frequency, Vtz - Peak Z axis vibration transmissibility.
Correlation and Regression
The total transmissibility, averaged for all frequency (VTz_avg), demonstrated moderate positive correlation to body mass composition, except for the Fat% (r = - 0.034). Deconstructing frequency at each experimental value also indicated non-significant correlation with body composition parameters.
The correlation between VTz_avg and waist size was significant (r=0.479). Univariate regression showed strong positive association with transmissibility (β=0.48, R2=0.23, p=0.02) and is presented in Table 4. The graphical presentation of positive association between waist size and total average vibration transmissibility (VTz_Avg) is given as univariate regression plot in Fig 7.
Item | Corre- lation | Fat% | Lean Mass% | DLW (kg) | Water % | BMI (kg/m2) | Bio-IMD (Ω) |
---|---|---|---|---|---|---|---|
VTz_avg (m/s2) | R | -0.03 | 0.22 | 0.16 | 0.20 | 0.22 | 0.03 |
Age (years) | Height (cm) | Weight (kg) | Waist (cm) | Hip (cm) | Sitting height (cm) | ||
R | 0.233 | 0.083 | 0.199 | 0.479 β= 0.48, R2=0.23, p=0.02 | 0.306 | 0.345 |
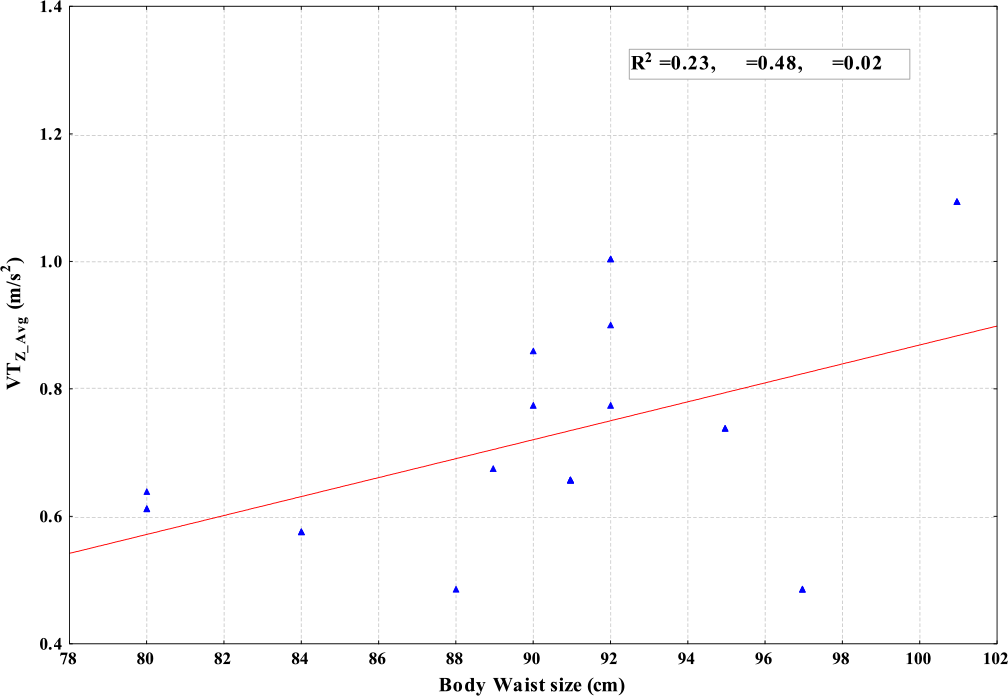
- Regression plot for Z-axis vibration transmissibility and waist size
However, inspecting relationship of transmission at each frequency, showed significant correlation with body parameters as given in Table 5. The waist size was positively correlated to vibration transmission at 4 Hz and 8 Hz with r = 0.42 and r = 0.54 respectively. Similarly, for sitting height, the relationship was significant at 6 Hz (r = 0.43) and 18 Hz (r = 0.46). For significant correlation results, simple and multiple regression analysis was carried out and results are given in Table 6. Waist size in sitting position had positive significant association with transmissibility at 4 Hz (β =0.429, R =0.184, p=0.041) and 8 Hz (β=0.542, R =0.294, p=0.008). The regression analysis predicted an increased transmissibility with increasing hip size at 6 Hz (β=0.430, R =0. 185, p=0.040). Sitting height was also positively associated with Z axis transmissibility at 6 Hz (β=0.524, R =0.274, p=0.010 and 18 Hz (β=0.461, R =0.213, p=0.027. However, multiple regression suppressed positive relationship between transmission to waist and sitting height together.
Items | Corr- elation | 4Hz_ Avg | 6Hz_ Avg | 8Hz_Avg | 18Hz_ Avg | 21 Hz_ Avg | |
---|---|---|---|---|---|---|---|
VTz (m/s2) | Fat % | r | -0.17 | 0.16 | -0.01 | 0.07 | 0.05 |
Lean mass% | 0.24 | 0.29 | 0.15 | 0.17 | -0.03 | ||
DLW (kg) | 0.09 | 0.35 | 0.16 | 0.16 | -0.08 | ||
Water % | 0.28 | 0.14 | 0.04 | 0.21 | 0.07 | ||
Bio-IMD (Ω) | 0.08 | 0.01 | -0.18 | 0.20 | 0.05 | ||
BMI (kg/m2) | 0.22 | 0.35 | 0.28 | 0.01 | -0.14 | ||
Age (years) | r | 0.18 | 0.36 | 0.01 | 0.29 | 0.24 | |
Height (cm) | -0.02 | 0.22 | -0.08 | 0.33 | 0.14 | ||
Weight (kg) | 0.14 | 0.37 | 0.14 | 0.21 | 0.00 | ||
Waist (cm) | 0.43 | 0.41 | 0.54 | 0.36 | 0.14 | ||
Hip (cm) | 0.32 | 0.43 | 0.29 | 0.10 | -0.06 | ||
St Ht (cm) | 0.27 | 0.52 | 0.06 | 0.46 | 0.27 |
Linear Regression | Bodyparameter (cm) | Correlation atfrequency | Ω | R2 | p-value |
---|---|---|---|---|---|
Waist |
4Hz_ Avg r = 0.43 |
0.429 | 0.184 | 0.041 | |
8Hz_ Avg | 0.542 | 0.294 | 0.008 | ||
Simple univariate | r = 0.54 | ||||
Hip |
6Hz_ Avg r = 0.43 |
0.430 | 0.185 | 0.040 | |
St Ht |
6Hz_ Avg r = 0.52 |
0.524 | 0.274 | 0.010 | |
18Hz_ Avg r = 0.46 |
0.461 | 0.213 | 0.027 | ||
Multiple univariate | Hip |
6Hz_ Avg rHip = 0.43 rSt Ht = 0.52 |
0.195 | 0.300 | 0.401 |
St Ht | 0.413 | 0.085 |
Discussion
Transmission of vibration to the body is a complicated phenomenon because of nonlinearities in the human musculoskeletal system. Analysis of the response to whole body vibration is challenging and subject to several confounding factors. There are substantial differences in individual transmissibility, which are affected by body posture, muscle activity, body segment weights and biomechanics. Accordingly, the propagation of whole body vibration is markedly influenced by nonlinearities in the body biomechanics and it is not possible to infer the peak value of the site-specific acceleration from the amplitude and frequency of sinusoidal vertical vibration using a simple theoretical relationship.
In-flight vibration measured in Cheetah and Pratap helicopters suggest that the intensity of vibration is predominant in the vertical Z-axis and fore & aft vibrations are very small [8]. In the present study, seat to head vibration transmissibility in seated posture was studied for Z-axis vertical vibrations.
Biodynamic Frequency Response
Human body can be considered a mechanical system that is interconnected by spring, mass and dampers. Its transmissibility property in seated posture and dependence on vibration frequency and body posture, is influenced by the stiffness and damping properties of head/neck, thigh, pelvis and torso. Human body response to frequency in seated posture was very well studied in our experimental approach. The measured value of seat to head transmissibility was definitively sensitive to low frequency bandwidth 4-21 Hz range.
Sitting Posture
Sitting posture in combination with vibration pressure at seat interface definitely affects the occupants’ comfort and performance in different ways. Depending on the design of the seat and seat suspension system, the vibration transmission may get influenced. Vertical oscillation of seated person at resonant frequencies above about 2Hz causes amplification of the vibration within the body. The first major resonance occurs at about 4-5Hz. The transmissibility of vertical vibrations to the head in present cases was maximum at 4Hz for all experimental conditions. It is been understood from experiments that change of posture from erect to slouch decreased the natural frequency of human body.
In a study by Clinton Rubin et al, standing position and extent of vibration transmitted to pelvis region was studied [9]. If mechanical signals of vibration can be effectively and non-invasively transmitted in the standing human to reach those sites of the skeleton at greatest risk of osteoporosis, such as the hip and lumbar spine, then vibration could be used as a unique, non-pharmacological intervention to prevent or reverse bone loss. With the subjects standing erect, transmissibility at the hip exceeded 100% for loading frequencies less than 20Hz, indicating a resonance. However, at frequencies more than 25Hz, transmissibility decreased to approximately 80% at the hip and spine. In relaxed stance, transmissibility decreased to 60%. With 20-degree knee flexion, transmissibility was reduced even further to approximately 30%. This also explains the result of the present study, where-in, in relaxed stance at 4, 6 and 8 Hz the transmissibility increased in sitting posture but for 18-21 Hz the transmissibility decreased in slouch posture as compared to erect posture (Fig 6).
Mechanical vibration was used to investigate the role of postural stability in case of patients with history of back pain and normal population. It was observed that on vibrating platform the postural from control strategy acquired by group having LBA was different from control group [10].
Head Supported Mass
HSM alone was not significant but in combination with posture and frequency it was significantly related to the transmission. Adding NVG and CW, indirectly increases the mass of the body and positive association was found with transmission. Research studies and analytical model tests have shown that the biodynamic response characteristics of the seated human body slightly increases with body mass. In our study also though the correlation was not significant, but it was positive with VTz at different study frequencies [11].
Anthropometry
The body weight ranged from 53-83kg. Even though the VTz_avg transmission and body weight were not correlated, the significant correlation at 6 and 8 Hz can be related to resonance and body mass composition that is related to body weight. Based on the analytical investigation conducted by Mostafa AM and Abbas W, it could be concluded that the change in human body's mass, pelvic stiffness and pelvic damping coefficient gives a remarkable change in biodynamic response behaviors of seated human body. It has direct proportionality to human body's mass and pelvic stiffness coefficient and inverse proportionality for pelvic damping coefficient. In our study also waist and hip size were found to have significant correlation with transmissibility in seated human being at vibration frequency of 4, 6 and 8 Hz. It was also observed that with increase in body weight the transmissibility increases [12], however in our study none of this relation was observed .
Body Mass Composition
The body mass composition (Fat, Lean mass, DLW, Water, BMI and Bio-IMD) did not show any significant correlation with the vibration transmissibility. In a study by Mythili S and Athisha G, significant relationship of BMI and BMD to leg bone acceleration was observed [13]. In our study, there was insignificant positive correlation of BMI to vibration transmissibility at 6 -18 Hz. This may be due to the fact that the mean age of the sample population was 20.17±6.64 years. At this young age it is rare to show increase in transmissibility due to weight or age related bone degeneration. Similarly, there was insignificant negative correlation with the fat%, but it indicated that fat attenuated the VTz_avg transmission of the vibration through torso and head. The understanding can be increased by categorizing the participants into, under weight, normal, over weight and obese category and in different age groups.
Correlation and Regression
There was insignificant correlation between anthropometry and VTz_avg. However, breakup for individual frequencies showed that the DLW (r=0.48) and BMI (r=0.51) had significant correlation at erect sitting position for 8 Hz and slouch posture with NVG at 6 Hz respectively. But these factors may not predict the transmissibility, as regression analysis showed insignificant association having values (β=0.194, R2=0.276, p=0.545) and (β =0.356, R2 =0.276, p = 0.273). It is also established that a specific pattern was not observed in correlating and associating the anthropometry and body mass composition to transmissibility. However, the univariate regression analysis showed significant association with age at 6Hz, for NVG-CW assembly. The vibration transmission increased at 6 Hz for NVG-CW assembly indicating its selectivity to frequency and HSM. Though the correlation of VTz was observed for DLW and BMI at 6 and 8 Hz, the regression analysis could not establish significant association.
Pain Questionnaire
The disability score (in %) was less than 20%, and fell in minimal disability category. The participants did not have any symptoms of pain. The results may be due to the fact that the volunteers who participated in the present study did not have any history of back pain or musculoskeletal injury. Vibration is most often considered an etiologic factor in low back pain as well as several other musculoskeletal and neurovestibular complications. But some latest studies in recent past, in animals, indicated that extremely low-level mechanical signals delivered to bone in the frequency range of 15 to 60 Hz can be strongly anabolic [7].
Transmission of vertical vibration to the ankle, knee, hip and spine was analyzed in a study by Juha K et al. Substantial amplification of peak acceleration occurred between 10 and 40 Hz for the ankle, 10 and 25 Hz for the knee, 10 and 20 Hz for the hip, and at 10 Hz for the spine [1]. This means that the site-specific peak accelerations can be multiples of that imposed at the vibration platform. Beyond these frequencies, the transmitted vibration power declined to 1/10th−1/1000th of the power delivered by the vibrating platform. In general, the transmitted accelerations were least attenuated at the ankle and most at the spine. Differences in transmissibility between subjects could be 10:1 or even more for some conditions, but for certain amplitudes and frequencies, the transmissibility was rather similar in all subjects.
Body mass is significantly associated with quad bike induced WBV (expressed as 1hrVDVZ) in a group of New Zealand rural workers [14]. Other intrinsic factors such as height, age and quad bike experience were not associated with vibration exposure. These results for body mass should be considered by others undertaking WBV research on small vehicle vibration exposures. Reduction of vibration exposures is considered an ergonomic intervention of importance in drivers of commercial vehicles. Attenuation of vibration by body mass alone may need to be factored into design of seating and suspension systems for small on-farm vehicles.
Studies at IAM
The vertical vibration transmissibility in seated subjects for an erect posture was found to be maximum in the frequency range 5-6 Hz. In our study both erect and slouch posture were studied and significant difference was found. In one particular study, it was found out that the rudder pedal operation gets maximally affected in the range of 3-7 Hz [15]. In the present study mechanical impedance was not studied.
Frequency dependent transmission characteristics and their validation through mechanical impedance was studied by Vyawahare et al [16]. It was observed that with increase in subject's weight the frequency of mechanical impedance decreased. The magnitude of mechanical impedance increased with increase in weight.
Vibration transmissibility at shoulder and thigh levels in upright seated individuals with respect to seat vibration was determined and also compared to the body weight. It was concluded that vibration intensity at thigh and shoulder levels of a seated upright subject is less than at the seat level [17].
Conclusion
In this paper, seat to head, Z–axis vibration transmissibility was successfully studied in a group of 23 male Indian volunteers of age group 20-39 years. We observed that vibration transmissibility was significantly influenced by the sitting posture and frequency. Although the head supported mass individually was insignificant, the transmission was observed to increase moderately after mounting NVG-CW assembly. The interactive effect of these experimental factors also influenced transmission significantly. Based on the experimental and statistical analytical investigation we demonstrated that there was no significant correlation of body composition parameters to vibration transmission in our study. It can also be concluded that the anthropometric parameters had strong association to vibration transmission at specific frequencies. Though the pain score in the present study was insignificant, the study would be important for understanding discomfort, fatigue, LBA and neck pain under environmental vibration as postural stability acquired by cases with LBA is different than normal cases.
Recommendations
Multiaxial vibration exposure based study may give more realistic approach to occupational vibrations. The study can be extended to Osteoporotic cases so that usage of setup in assessing the quality of bone can be proved. Further research investigation for correlation to bone mineral density and vitamin D to vibration transmission is recommended.
Acknowledgement
The authors thank all volunteers for their whole hearted participation. The help by Sqn Ldr B Bhowmick is duly acknowledged.
References
- Transmission of vertical whole body vibration to the human body. J Bone Miner Res. 2008;23(8):1318-25.
- [Google Scholar]
- Head and helmet biodynamics and tracking performance in vibration environments. Aviat Space Environ Med. 2006;77(4):388-97.
- [Google Scholar]
- Physiological effects of night vision goggle counterweights on neck musculature of military helicopter pilots. Mil Med. 2007;172(8):864.
- [Google Scholar]
- Seated body apparent mass response to vertical whole body vibration: gender and anthropometric effects. Int J Ind Ergonom. 2013;43(4):375-91.
- [Google Scholar]
- Lumbar back muscle activity of helicopter pilots and whole-body vibration. J. Biomech. 2001;34(10):1309-15.
- [Google Scholar]
- The Predictors of Low Back Pain in Helicopter Pilots. Gazi Medical Journal. 2015;26(1):15-18.
- [Google Scholar]
- In-flight measurement of vibration levels at seat and floor of Chetak and Pratap helicopters. Ind J Aerospace Med. 1996;40(1):29-35.
- [Google Scholar]
- Chris Fritton, Marianne Magnusson, Tommy Hansson and Kenneth McLeod Transmissibility of 15-Hertz to 35-Hertz Vibrations to the Human Hip and Lumbar Spine: Determining the Physiologic Feasibility of Delivering Low-Level Anabolic Mechanical Stimuli to Skeletal Regions at Greatest Risk of Fracture Because of Osteoporosis. Spine. 2003;28(3):2621-7.
- [Google Scholar]
- The Effect of Inspiratory Muscles Fatigue on Postural Control in People With and Without Recurrent Low Back Pain. Spine. 2010;35(10):1088-94.
- [Google Scholar]
- Transmissibility and DPMI analysis of the seated posture of human under low frequency vibration. Mechanical and industrial Engineering Department Indian Institute of Technology, Roorkee.
- [Google Scholar]
- Prediction of Biodynamic Response of the Seated Human Body, using Artificial Intelligence Technique. Int J Eng. 2011;4(6):491-501.
- [Google Scholar]
- Association of body mass index with bone acceleration on impulse response in men and women of south indian population Biomed. Res. 2014;25(4):471-475.
- [Google Scholar]
- The Influence of Body Mass on Whole-Body Vibration: A Quad-Bike Field Study. TOERGJ. 2011;4:1-9.
- [Google Scholar]
- Vibration biodynamics studies at IAM : An overview. Ind J Aerospace Med. 1994;38(2, spl.iss.1):166-9.
- [Google Scholar]
- Performance on hand and foot operated controls under low frequency vibration. Aviation Medicine. 1986;30(1):24-32.
- [Google Scholar]
- Vibration transmissibility in seated subjects. Aviation Medicine. 1984;28(2):162-170.
- [Google Scholar]