Translate this page into:
Colour vision standards: Past, present, and future
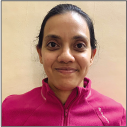
*Corresponding author: Dr S Dinakar, MBBS, MD (Aerospace Medicine), Department of Acceleration Physiology & Spatial Orientation, Institute of Aerospace Medicine IAF, Vimanapura, Bengaluru - 560 017, Karnataka, India. dinakarsneha@gmail.com
-
Received: ,
Accepted: ,
How to cite this article: Agarwal A, Dinakar S, Tripathy NK, Sharma V, Joshi S, Lagisetti SD. Colour vision standards: Past, present, and future. Indian J Aerosp Med 2020;64(2):93-9.
Abstract
Historically, signal lights (red-green-amber) were used in shipping, rail, and road transportation. This colour schema continued in the aviation industry too. However, automation has taken over aviation sector with electronic maps and colour-coded multifunction displays. Despite sweeping changes seen in the use of colour coding in aviation, there is little change in colour vision standards and in the way colour vision testing is done for the aircrew, military and civil. The changing needs of aviation dictate that renewing the standards is necessary. Furthermore, the new standards will dictate aircraft design, and hence, it is mandatory that they remain current for the next 50 years or so. It becomes necessary to understand the role colour vision plays in the modern cockpit and suggest the colour vision standards accordingly. In the same breath, it is important to understand the evolution of colour vision testing and colour theories, so as to develop or adopt a more suitable test for the changing aviation scenario.
Keywords
Multifunctional display
Colour vision standards
Colour vision testing
Aviation
Aircraft design
INTRODUCTION
Indian aviation, military and civil, use colour vision standards devised in the 1940s and borrowed from the RAF. The changing needs of aviation dictate that renewing the standards is necessary. Furthermore, the new standards will dictate aircraft design, and hence, it is mandatory that they remain current for the next 50 years or so. It is thus required to understand in detail colour vision, what various tests measure and what standards we should apply. This review article is aimed at addressing these issues considering military and civil aircrew as a single entity. The reason why there should not be a difference in standards between them is brought out toward the end of this review. The service providers such as the air traffic and radar controllers, and maintenance crew form part of the Indian aviation. It needs to be mentioned that the article deals with colour vision standards, the need for adopting them, and the methods for testing them, rather than defining a particular test.
Historically, signal lights, and the road, rail, and shipping accidents attributable to colour vision defects, drove the need for testing colour vision. For a brief period, automation and better navigation aids had reduced the requirement of colour vision.[1,2] However, with the advent of electronic maps and colour-coded multifunction displays (MFDs), the need for colour vision is back. Furthermore, there is a shift from the requirement to detect “Signal Colour” to “Appropriate Colour Vision.” Indian aviation uses Ishihara’s pseudoisochromatic plates (PIPs) as a screening test, even though this does not test for defective blue vision.[3] The Martin Lantern test (MLT), designed in 1939, tests only for white, signal red, and signal green.[4] Clearly, this testing is inadequate for modern day MFDs, which can typically generate 16 million colour.[1,2]
The International Civil Aviation Organization (ICAO) has given colour vision requirements, which may be interpreted by the member states.[5,6] Since the ICAO does not specify any particular test, every aviation agency applies its own tests to assess the standards set by the ICAO. This onerous task requires an immaculate understanding of colour vision, its use, components, and detection so that standards and testing evolve adequately.
THE USE OF COLOUR VISION
Colour coding enhances information gathering and assimilation, while decreasing fatigue. The time needed to locate or count colour-coded objects are shorter. The application of colour coding has been described by Cole in 1972.[1,2,7] The colour is essentially applied in three different ways; Connotative: known simply as signaling, it is used effectively for critical areas such as signal lights, PAPI lights, and undercarriage indications (three greens). Denotative: also known as colour coding, it is used to organize displays. The denotative colour of rivers on a map is blue and roads are black, so a single glance can indicate a river as being different from a road. Similarly, cross-border territory indicated in red will avoid a possible border transgression, which would be impossible on a monochromatic map. Esthetic: Esthetics generate mood and have been used effectively in the military, like Napoleon’s red coat, worn so that blood would not show in battle. Colour camouflage is used on all military machines as well as airfields, while display aircraft is painted in striking colours.
PERCEPTION OF COLOUR AND ITS EFFECTS ON TESTING
Isaac Newton in his 1704 publication “Opticks” brought out that white light was composed of several colours that could be split using a prism.[8] In 1801, Young proved the wave theory of light in the Bakerian lecture.[9] Helmholtz expanded Young’s ideas and proposed that the eye had three kinds of photoreceptors that perceived light of short, medium, and long wavelengths. The revolutionary idea that our perception of millions of colours depends on just three distinct colour receptors is called the “Young–Helmholtz Trichromatic Theory” (1860).[10] The understanding of the trichromatic theory was augmented by Hering in 1878 who hypothesized that the trichromatic signals from the cones fed into two opponent classes for processing – called the “Opponent-Process Theory” and has been described in more detail below.[10,11] Schultze dissected retinae of mammals, fish, and birds in 1866 and concluded that cones mediated chromatic vision and rods mediated achromatic or night vision.[10] The understanding of psychophysiological aspects of colour vision is still expanding.
Colour is perceived using three characteristics, namely, hue, saturation (or chroma), and brightness (or lightness).[11] Hue is defined as the attribute of colours that permit them to be classed as red, yellow, green, and blue and is a function of the wavelength of light being emitted/reflected. The perception may be modified by the hue of surrounding objects as well as after shadows. Saturation (or chroma) indicates the chromatic purity, namely, freedom from dilution with white. It reflects the vividness or dullness of a colour and indicates the degree of absence of whiteness, that is, 100% saturated means that there is no addition of white to the hue. Brightness is defined as the attribute of light source colours by which emitted light is ordered continuously from light to dark in correlation with its intensity.[10,11]
Physiology of the human visual system
Human visual content and perception have changed. Earlier, humans spent long times in the darkness of caves and jungles with no artificial light. The primary mode of vision was scotopic, using the rods which form 95% of the photoreceptors (100–120 million). By contrast, now almost all our vision is cone (photopic) based even though the cones make up only 5% of the photoreceptors in the retina (3–5 million cones). The cones are densely packed in the fovea, while rods are located almost exclusively in the periphery of the retina. The foveola is rod free and the visual acuity relies on as few as 100,000 cones (or 0.1% of total number of photoreceptors).[12,13]
The three kinds of cones active in the human visual system are the short-wavelength (S) cones with a maximum response at 450 nm (violet-blue), medium-wavelength (M) cones with a maximum response at 530 nm (green), and long-wavelength (L) cones with a maximum response at 560 nm (greenish-yellow).[14] In the center – most section of the fovea, the foveola, S cones are virtually absent, reducing our sensitivity to small blue objects.
The photoreceptors transmit neural responses through the bipolar, horizontal, and amacrine cells, which combine and compare the responses from individual photoreceptors before transmitting the signals to the retinal ganglia cells. For approximately 120 million photoreceptors, there are only about 1.6 million ganglion cells, that is, in a ratio of 1:100. While 60–100 rods converge onto a single ganglion cell, only two cones do so, improving the visual acuity of cones. This ratio is very high in the periphery and less near the fovea. Close to the fovea the rods and cones also become more slender. These effects progressively increase the acuity of vision in the central retina. In the central fovea, there are only about 35,000 slender cones and no rods, with each cone being represented in a ratio of 1:1, resulting in the maximum possible visual acuity in the central retina.[14-16]
Influence of the opponent theory on design of colour vision tests
Stimulation of cones by electromagnetic energy in the visual range generates signals that are perceived as colour vision. Three cones that respond best to yellowish-green (L), green (M), and indigo (S) can perceive 16 million colours, and hue, saturation, and brightness for each. This seemingly impossible feat is explained by the opponent-process theory.[15,17] This is achieved through four interpretation paradigms; (a) brightness is interpreted using the combined stimulation of the M and L cones. This is also known as the white-black channel. (b) Yellowness is interpreted by the difference between “Brightness” and S cones, that is, if both M and L are strong and S is weak, it is interpreted as yellow, while if all three are strong, it is interpreted as white. (c) If the S cones are relatively stronger than the M and L, it is interpreted as blue. (d) The difference between M and L is interpreted as green-red.[11] The above would indicate that light with the same blue hue, with a slight change in the brightness could result in “colours” looking yellow or blue. Similarly, a slight change in S cones would look like blue or dark or any other bizarre combination. This would translate specifically to tests used in routine, as under:
Pseudo-Isochromatic Plates (PIP)
PIP makes use of varying hue and saturation to detect colour vision defects. The test is to be done in “adequate light.” The change in lighting could change the hues due to the opponent process. This needs to be, but seldom is, understood by medical examiners.[11]
Lantern tests
Lantern tests have an inherent light source, rather than the reflected light tests like PIP tests. This is an advantage. The MLT originally used various filters to vary hues, saturation, and brightness, but is now reduced to signal colours only.[4,10,11]
Computer-based tests
Computers have the advantage of providing up to 16 million colours and very subtle changes in brightness.[18] This makes computers the ideal platform for modern colour vision tests. These have been explored further below.
Evolution of colour vision testing
Signal lights were introduced in shipping and later in rail transport in the early 19th century. The colours used were red, green, and white.[19,20] A train accident occurred at Lagerlunda in Sweden on November 15, 1875, where two trains collided on a single-line track leaving nine dead.[21] Holmgren, a professor of physiology, suggested the possibility of the accident having resulted from colour blindness. His tests on all employees indicated colour deficiency in 4.8% of the personnel, including a stationmaster and an engineer. This spurred the development of colour vision tests between 1870 and 1930.[7,19,20,22] In aviation, according to Tredici (1972), the United States Air Force (USAF) devised colour standards in 1918.[7]
Scientists were aware of colour vision defects since much earlier. One major development came in 1837, when Seebeck introduced matching of 300 hues of paper, rather than naming them,[23] thus obviating lexical semantics. Seebeck’s work allowed differentiation of red-green blindness and also the development of a severity scale. Holmgren adopted and modified this test in 1877 using skeins of wool. The Holmgren wool test was widely used and even commercially available more than 100 years later.[19,23]
Two major developments in colour vision testing came in the last quarter of the 19th century. Stilling, a German ophthalmologist, published the first painted set of PIP in 1878, which filled an important void, and was immediately commercialized. It had 14 successive editions until the author’s death in 1915, after which it continued with newer editions modified by successive authors.[7,19,22,23] The second major development was by Rayleigh (1842–1919) who demonstrated the peculiarity of human trichromatic/ opponent vision that forms the basis of all advanced colour vision testing. He demonstrated that mixing of red-green lights could be not be differentiated from yellow. This match known as Rayleigh match is basis of all modern anomaloscopes. This also helped define dichromatism and anomalous trichromatism.[7,19,22,23]
AVAILABLE COLOUR VISION TESTS
Pseudoisochromatic tests
PIP tests were developed based on observation wherein the colours were selected randomly and plates showing diagnostic efficiency were retained. The plate design is complicated by factors such as form, size, glossiness, texture, and glitter. Stilling applied information obtained from two colour-defective assistants (one red-green blind, the other blue-yellow blind). He used this information ingenuously, in conjunction with the opponent theory to hand paint a series of plates in which figures composed of one set of variegated colour dots appeared on a background of corresponding confusion colours. For instance, he used red-orange dots on a dull yellow background and yellow-green dots on an orange-brown background to detect red-green deficiencies, and pale blue dots on a pale yellow-green background to detect yellow-blue deficiencies.[11,19,22,23]
Soon after the outbreak of WW II, Japanese Ophthalmologist Dr. Shinobu Ishihara was asked by the Japanese Army to develop a colour vision test for its conscripts. He expanded Stilling’s work to achieve more technical results. Using standard camouflage technique, he used a configuration of dots to disintegrate characters, if they could not be identified on the basis of hue.[5,10,23,24] He was the first to create a hierarchy of plates that could be divided as follows: Demonstration plates that were read by all subjects, normal or pathological, vanishing plates that could be read by normal subjects but not read colour defective subjects, transformation plates that are read differently by normal and colour defective subjects, hidden plates that could not be read by normal subjects, but read by colour defective subjects, qualitative plates that indicated the type of the dyschromatopsia, and quantitative diagnostic plates that indicated the severity of the dyschromatopsia.
Ishihara hand painted each plate in watercolour with Japanese hiragana characters and completed his work in 1916. The test was reconfigured in 1921 for use outside Japan by replacing hiragana characters with numerical designs and sent to universities and ophthalmologists across the world.[3] This led to a wide acceptance and its adoption for assessing merchant seamen, railway workers, and later navy and air force personnel. Despite its inability to test for blue blindness, it remains the most widely used test in the field.[3]
At present, there are many other plate tests such as Stilling-Velhagen, Dvorine, Bostrom and Kugelberg, and Hardy, Rand, and Rittler. All make use of the four basic plate designs invented by Ishihara, namely, demonstrative, vanishing, transformation, and hidden.[5,19] Some modern tests such as the Tokyo Medical College Test, test for blue blindness, can quantify the defect better.[25] However, as has been brought out earlier in the discussion on the opponent theory, a change in illumination can change the hues perceived on a plate. This may not be understood well enough even by experienced examiners and may be a frequent cause for misinterpretation of the tests.[19,25]
The low cost, mass production, and rapid administration of PIP tests make them an ideal screening tool. However, the accuracy of the results depends on the interpretation. Furthermore, printing differences between different lots of plates may lead to errors, as can changes in illumination. These plates can be memorized, leading to cheating in enthusiastic applicants.[26] A consequence of these difficulties is that there are no international pass-fail criteria for PIP tests.
Lantern tests
The signals for both shipping and railways in the late 19th and early 20th centuries consisted of lanterns with coloured filters. Hence, it was logical to use similar, standardized lanterns to assess colour vision defects in operators. The original Board of Trade Lanterns (1912) showed “flashed ruby” and “signal green” lights to the observer, either singly or in pairs from a fixed distance of 20 ft. The construction was simple.
There were small and large apertures (controlled by circular diaphragms), the light was furnished by an oil lamp and the colour filters consisted of specimens of glasses with other neutral filters.[4] Over the years and with the availability of electric power, the oil lamp was substituted with an electric light by Martin (1939).[6]
Several different models of lanterns are available: Giles-Archer, Holmes-Wright, Royal Canadian Air Force, Optec-900, Edridge-Green, Martin’s, Sloan Colour Threshold Tester, and Farnsworth Lantern. Lantern tests are easy to administer. Their value lies in their simulation of the working condition. Lantern tests do not specifically screen for colour defect, although it is expected that colour-defective observers will not perform as observers with normal colour vision.[1,2,7,19,27]
In view of this, it would be worth reiterating that lantern tests are quite inadequate for any testing other than for signal colours. Furthermore, they have little to recommend themselves above the PIP tests. Since modern-day cockpits employ multiple colours different from signal colours, lantern tests have no place in colour vision testing for modern aviation.[28]
Arrangement tests
Arrangement tests involve arrangement of a set of coloured objects according to their similarity in colour. Examples are the Farnsworth–Munsell 100 hue test and its shortened version, the Farnsworth–Munsell dichotomous D-15 test, that consists of a series of coloured disks and colour vision defects are diagnosed by incorrect order of disks. A numeric score can be calculated and displayed on a polar graph. Age norms have been prepared giving the range of the expected total error scores for an unselected population as well as the expected intereye variability.[28,29] Fineness of discrimination can be added using more hues as in the 100 hue test, as against the D-15. Similar tests may be used to test lightness discrimination (Verriest’s lightness discrimination test) or to test saturation discrimination (Sahlgren’s saturation test and Lanthony new colour test).[29,30]
Observers with congenital colour vision deficiencies make characteristic errors on arrangement tests because their chromatic discrimination ability is lost on particular axes in chromaticity space. Discrimination loss in acquired colour vision defects is more variable. Different hues assess defects of S, M, and L cones. The partitioned scores can be examined to determine whether an acquired colour vision defect causes a particular type of discrimination loss, that is, the S or L/M systems.[29,30]
Anomaloscope
The principle of the anomaloscope is the fact shown by Rayleigh that the human eye cannot distinguish between pure yellow (from the sodium line, 589 nm), and the mixture of red (from lithium line, 537 nm) and a green (from thallium line, 665 nm), in a certain proportion.[31] If the brightness of yellow is now changed, the normal eye cannot adjust the red/green to achieve the new brightness. However, a defective eye can still make the match (as it cannot see the relative red-green).[24,31]
Rayleigh’s initial equation became the gold standard congenital red-green deficiencies. However, more complex deficiencies affecting the blue-yellow axis spawned other colour equations as well, for example, Engelking (1925) allowed the equation blue 470 nm + green 517 nm = cyan 490 nm. Several other instruments based on various equations followed (Trendelenburg, Pickford, Jack Moreland).[24,31] However, their calibration and use require a high degree of skill.[19] Interpretation of results is time consuming and requires expertise. These issues preclude mass use of anomaloscopes, despite them being the gold standard for colour vision defect detection and grading.
Cone contrast test (CCT)
The CCT by Dr. Jeff C. Rabin of the USAFSAM is unique in that it pays as much attention to the background as the letters to be identified. Second, the CCT does not test for all the myriad colours, but concentrates on stimulating individual cone type populations, that is, L-cone, M-cone, and S-cone.[32,33]
The opponent theory has been explained in detail above. The CCT has been designed to isolate the cone-opponent mechanisms by designing test stimuli that change the contrast to the cone-opponent mechanisms with minimal change in achromatic contrast or brightness. In addition, random achromatic contrast is often added to the background to further limit detection by the achromatic mechanism.[32-34] The cone contrast is computed as the difference between letter and background divided by background multiplied by 100: Cone contrast The test is conducted monocularly in a dark room at 1 m after adequate refraction. The test begins with a random series of 20 reddish letters (L), followed by greenish letters (M), and then violet letters (S) progressing from most visible down to least visible. The letter appears for a duration of 1.0–1.6 s (duration increases as contrast decreases) followed by an intertrial interval (grey field) of equal duration.[32,33] The log contrast sensitivity scores are normalized to an intuitive 100 point scale so that each letter that is read correctly counts as 5 points, making the max score 100 and the minimum score 0.
THE QUEST FOR THE IDEAL COLOUR VISION TEST: STANDARDS FOR THE INDIAN SCENARIO
Peculiarities of the Indian Scenario
The Indian scenario is different from other countries, which make designing of colour vision tests easier to some extent. These factors are Common Regulator: The Director General of Medical Services for the Air Force is the advisor for medical standards for all flying in India, military, as well as civil. Common Airfields: Civil flying in India happens from defense airfields and vice versa. This requires common standards for all safety critical issues, including colour vision. Common Airspace: The Govt. of India has removed restrictions from civil flights in military airspace, requiring commonality between standards. Expansion of Civil Flying: Civil flying in India is expanding exponentially. As new aircraft is being acquired, it is possible to influence manufacturers to adhere to regulatory requirements specific to India. Indigenous Designing of Aircraft: The Indian aircraft industry stands at a cusp where it is about to design and fly more and more indigenous aircraft, both military and civil. There is thus the need for a common standard that the industry can follow, to assure safety.
Characteristics of an ideal colour vision test
As has been brought out above, a colour vision test introduced today will need to be compatible with today’s technology and future ready. The following are the envisaged characteristics for an ideal colour vision test:
Longevity
Pilots selected today would be in service for four decades, as will the aircraft. Hence, the standards applied today need to be long lasting.
Inherent light source
Keeping in mind, the inaccuracies injected by the use of reflected light, the test should have an inherent light source.
Employing cone contrast
The discussion above indicates that the use of opponent theory using cone contrast is perhaps the most scientific assessment technique for a modern colour vision test.
Screening versus grading
The same test should allow for a rapid screening sequence and a more detailed grading of severity of colour deficiency.
Automatic calibration
The apparatus should be able to autocalibrate, using ISO or BIS or any other standardized calibration standards for luminance, hue, saturation, and brightness. Similarly, the ambient illumination also needs to be specified and calibrated.
Pre-manufactured versus generic test
The lure of a pre-manufactured test apparatus is ever present as it is easier to standardize and calibrate, free from idiosyncrasies of monitors and graphic cards, and can be easily distributed. The apprehension is that it may end up like the Martin Lantern, unavailable a few years later, starting the quest for a new device. This interferes with the first principle of longevity.
Sensitivity
The need for high sensitivity in the aviation scenario is easily understood. It is felt that if the above principles are applied, an extremely sensitive test may be possible.
Specificity
Any test or testing standard is always a trade-off between sensitivity and specificity. Since the said test would be an entry-level elect out standard for aviation, a lower specificity may be acceptable.
CONCLUSION
Aviation has moved much beyond signal colours with the use of MFDs and large area displays. There has been a rapid change over the past 40–50 years. It is now important to diagnose colour vision deficit and grade severity, as this may impact not only safety but also efficiency. A computer-based test with the underlying principle of cone contrast appears the most pertinent at this time. Agencies should look forward to induct a test that is more evidence based and suitable to the aviation industry. Such a test should be future ready, capable of both screening and grading, generic rather than pre-manufactured, autocalibrating, and should have a very high sensitivity even at the cost of specificity.
Declaration of patient consent
Patient’s consent not required as there are no patients in this study.
Financial support and sponsorship
Nil.
Conflicts of interest
There are no conflicts of interest. NK Tripathy is the editor of this journal. He does not have any competing interest.
References
- Are colour vision standards justified for the transport industry? Ophthalmic Physiol Opt. 1988;8:257-74.
- [CrossRef] [PubMed] [Google Scholar]
- Colour Vision. Section 11.8 In: Ophthalmology. Manual of Civil Aviation Medicine (3rd ed). Canada: International Civil Aviation Organization; 2012. p. :49-53.
- [Google Scholar]
- Minimum Colour Vision Requirements for Professional Flight Crew. 2020. Part 1: CAA Paper No. 2006/04. Available from: http://www.publicapps.caa.co.uk/modalapplication.aspx?appid=11&mode=detail&id=2407 [Last accessed on 2020 May 25]
- [Google Scholar]
- John Dalton's Eyes: A History of the Eye and Colour Vision. 2017. Part Two: Hektoen International. Chicago: Electronically Publishing; Available from: https://www.hekint.org/2017/01/22/john-daltons-eyes-a-history-of-theeyeand-color-vision-part-two [Last accessed on 2020 Apr 28]
- [Google Scholar]
- A standardized lantern for testing colour vision. Br J Ophthalmol. 1939;23:1-20.
- [CrossRef] [PubMed] [Google Scholar]
- Origins of colour vision standards within the transport industry. Ophthalmic Physiol Opt. 1986;6:369-75.
- [CrossRef] [PubMed] [Google Scholar]
- Opticks: Or, a Treatise of the Reflections, Refractions, Inflexions and Colours of Light. London: Printed for Sam, Smith, and Benj. Walford, Printers to the Royal Society; p. :1704.
- [CrossRef] [Google Scholar]
- The Bakerian lecture, On the theory of light and colours. Philos Trans R Soc. 1997;92:12-48.
- [CrossRef] [Google Scholar]
- Colour perception In: Physics Today. Maryland: American Institute of Physics; 1992. p. :23-9.
- [CrossRef] [Google Scholar]
- Why are rods more sensitive than cones? J Physiol. 2016;594:5415-26.
- [CrossRef] [PubMed] [Google Scholar]
- Evolution of eyes In: Encyclopaedia Britannica. Chicago: Encyclopaedia Britannica, Inc.; 2020. Available from: https://www.britannica.com/science/photoreception/evolution-of-eyes [Last accessed on 2020 Jun 18]
- [Google Scholar]
- Recent developments in the physiology of colour vision. Trends Neurosci. 1984;7:243-8.
- [CrossRef] [Google Scholar]
- Human visual pigments: Microspectrophotometric results from the eyes of seven persons. Proc R Soc Lond B Biol Sci. 1983;220:115-30.
- [CrossRef] [PubMed] [Google Scholar]
- An opponent process theory of color vision. Psychol Rev. 1957;64:384-404.
- [CrossRef] [PubMed] [Google Scholar]
- Visualization systems for multidimensional CLSM images In: Pawley JB, ed. Handbook of Biological Confocal Microscopy, Ch. 14. New York: Springer; 2007. p. :211-54.
- [CrossRef] [Google Scholar]
- National Research Council (US) Committee on Vision Washington, DC: National Academies Press; 1981.
- [Google Scholar]
- The Lagerlunda collision and the introduction of color vision testing. Surv Ophthalmol. 2012;57:178-94.
- [CrossRef] [PubMed] [Google Scholar]
- The origins of modern color vision In: The Science of Color, Ch. 1. Amsterdam: Elsevier; 2008. p. :1-36.
- [Google Scholar]
- Color Blindness Tests San Francisco: Electronically Published, Word Press; 2010. Available from: https://www.color-blindness.com/2010/03/23/color-blindness-tests [Last accessed on 2020 Jul 07]
- [Google Scholar]
- Dalton finally came In: The History of Color Blindness. Amsterdam: Kugler; 2013. p. :11-30.
- [Google Scholar]
- Color blindness and military fitness for duty: A new look at old standards. Mil Med. 2010;175:84-5.
- [CrossRef] [PubMed] [Google Scholar]
- The farnsworth-munsell 100-hue and dichotomous tests for color vision. J Opt Soc Am. 1943;33:568-78.
- [CrossRef] [Google Scholar]
- Nagel's anomaloscope for testing color vision. Trans Am Ophthalmol Soc. 1915;14:161-5.
- [Google Scholar]
- Cone-specific measures of human color vision. Invest Ophthalmol Vis Sci. 1996;37:2771-4.
- [Google Scholar]
- Rapid quantification of color vision: The cone contrast test. Invest Ophthalmol Vis Sci. 2011;52:816-20.
- [CrossRef] [PubMed] [Google Scholar]
- Operational Based Vision Assessment Cone Contrast Test: Description and Operation. 2016
- [CrossRef] [Google Scholar]