Translate this page into:
Retro-fitment of an oxygen system in a transport aircraft of Indian Air Force: Decompression chamber trials to assess system functionality
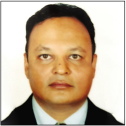
*Corresponding author: Ranjan Sarkar, Department of High Altitude Physiology and Hyperbaric Medicine, Institute of Aerospace Medicine, Indian Air Force, Bengaluru, Karnataka, India. sarkar.riddhi@gmail.com
-
Received: ,
Accepted: ,
How to cite this article: Raju AVK, Sarkar R, Joshi VV, Tripathy NK. Retro-fitment of an oxygen system in a transport aircraft of Indian Air Force: Decompression chamber trials to assess system functionality. Indian J Aerosp Med 2022;66:43-7.
Abstract
The aeromedical concerns of sudden onset hypoxia and rapid decompression are an omnipresent threat to flight safety when flying over 10,000 ft. The concept of Time of Useful Consciousness (TUC) provides the aircrew a window to correct his predicament. The issues of hypoxia are mitigated in transport aircraft with cabin pressurization. However, the requirement of an oxygen system in these aircraft is considered essential in an emergency in case of cabin failure of cabin pressurization or rapid decompression, despite adequate TUC, as it provides immediate succor from anxiety, and subtle performance decrements, especially in VIP carriage or casevac roles. With this background, a requirement for retro-fitment of a functional oxygen system of a small transport ac of Indo-German origin into a pressurized medium-lift cargo aircraft of British Origin was envisaged. Toward this, ground-based simulator trials were conducted on the oxygen system to assess its functionality before undertaking flight trials in the aircraft. The assessment consisted of the ground trial of the test oxygen system in the Explosive Decompression Chamber with three experiment protocols. The experimentation involved exposure to a simulated altitude of 15,000 ft for 25 min and rapid decompression from 7000 to 15,000 ft. Physiological parameters, endurance, and flow of oxygen in the system were evaluated. The trial results indicated that the physiological parameters (heart rate, respiratory rate, oxygen saturation [SpO2], and end-tidal carbon dioxide [EtCO2]) were within normal limits during the entire duration of exposure to 15,000 ft and decompression from 7000 ft to 15,000 ft. In addition, the physiological parameters were also within normal limits during exposure to 1 min of simulated hyperventilation. Neither any subjective or objective symptoms of hypoxia were observed nor any appreciation of increased breathing resistance reported by the subjects. The endurance of the system in terms of delivering continuous oxygen flow at 15,000 ft was assessed to be 73 min. From the trials, it was inferred that the oxygen system in a single crew configuration could be used satisfactorily up to the operational ceiling altitude specified for the subject aircraft.
Keywords
Hypoxia
Rapid decompression
Oxygen system
Explosive decompression chamber
INTRODUCTION
The IAF operates various transport aircraft, each with its unique roles and operational characteristics. Some of these are in the IAF inventory for more than 3–4 decades and are still actively used as a troop and cargo carriers flying at altitudes above 15,000 ft, in a pressurized environment without a functional oxygen system, whereas there are unpressurized aircraft operating in much lower altitudes with a functional oxygen system. The aeromedical concerns of sudden onset hypoxia and rapid decompression are well established and remain an omnipresent threat in aircraft flying over 10,000 ft.[1] The concept of Time of Useful Consciousness (TUC) provides the aircrew an opportunity to correct his/her predicament and at 15,000 ft is more than 45 min and theoretically adequate. However, this is considerably shorter at a given altitude when hypoxia is induced by rapid decompression rather than by slow ascent.[1] There are also large individual variations of TUC due to various factors such as experience and training of aircrew, physical fitness standards, pulmonary response to hypoxia, and previous experience of hypoxia[1] and even mild decrements in performance are highly undesirable in aircrew while flying an aircraft in VIP or casevac configurations. This gains even more operational significance as, at about 15,000 ft, the ability to perform the skilled task is impaired without the awareness of the aircrew, despite having an adequate TUC.[1] With this background, it became imperative to retrofit a functional oxygen system of a small transport ac of Indo-German origin into a pressurized medium-lift cargo aircraft of British Origin (without a functional oxygen system), flying at an operational ceiling of 15,000 ft and arm its aircrew with a crew oxygen system.
The small twin-turboprop aircraft of Indo-German origin has a constant flow portable oxygen system comprising two identical sets of oxygen supply equipment which provides the pilot and copilot with the same quantity of oxygen at all operating altitudes. In terms of endurance, the oxygen carried by one main cylinder is sufficient to provide 52 min of a continuous flow of oxygen for one pilot (26 min each, for two aircrews). The regulator provides a continuous flow of 2.5 LPM from ground level and it cannot be regulated. The system needs to be removed from the aircraft to be charged from a ground source. The oxygen system is used only during smoke and fire hazard. This oxygen system was considered for retro fitment in the pressurized transport aircraft as an adjunct life support device during emergency get down procedure in case of cabin pressurization or during smoke or fire hazard. Toward this, ground trials of the system were carried out in the Explosive Decompression Chamber (EDC) at the Institute of Aerospace Medicine (IAM), Indian Air Force.
SCOPE, AIM, AND OBJECTIVES
The scope of the trials was limited to ground-based simulator trials involving human subjects to assess the suitability of the subject oxygen system as an emergency oxygen system. The aim was to assess the suitability of the oxygen system up to 15,000 ft and during decompression from 7000 to 15,000 ft. The objectives were to check the ability of the oxygen system to provide:-
Adequate oxygen to maintain satisfactory SpO2 during exposure to 15,000 ft and 30 s after decompression from 7000 to 15,000 ft
To provide an adequate flow of oxygen during hyperventilation on exposure to 15,000 ft and the following decompression from 7000 ft to 15,000 ft.
To assess the endurance capability of fully charged oxygen bottles at 15,000 ft with normal breathing.
TRIAL METHODOLOGY
Equipment assembly
The equipment used in the trials was as follows:-
01 × fully serviceable and fully charged (up to 1800 psi) portable oxygen system of the test article [Figure 1]
Finger SpO2 monitor for monitoring blood oxygen saturation
EtCO2 monitor for monitoring EtCO2 and respiratory rate
EDC at IAM IAF.

- Subject with portable oxygen system.
Trial protocol
The trials were conducted in the EDC at the Dept. of HAP & HM, IAM. A healthy male volunteer participated in the trial [Figure 2]. A Finger SpO2 monitor was used for monitoring blood oxygen saturation (SpO2), EtCO2 monitor was used for monitoring EtCO2 and respiratory rate. The simulator trials involved three experimentations. The first two experiments were carried out on a Dornier Oxygen system with a German make bottle. Experiment No. 1 involved exposure to an altitude of 15,000 ft for 25 min. In the second experiment, decompression was simulated from 7000 to 15,000 ft. The ability of the oxygen system to provide adequate oxygen to maintain oxygen saturation was assessed. In addition, the adequacy of the oxygen system to meet the increasing flow requirements during hyperventilation was assessed during both experiments. In the third experiment, the endurance capability of the oxygen bottle was assessed at 15,000 ft altitude with the subject breathing normally.
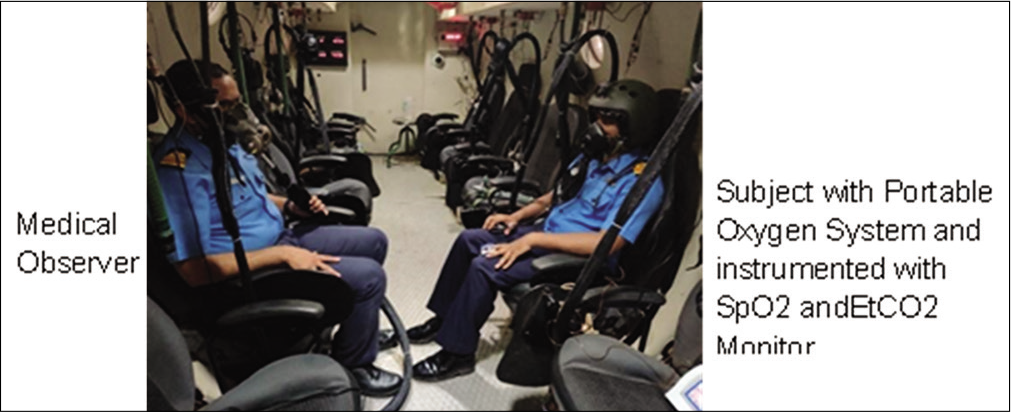
- Subject and medical observer in the explosive decompression chamber.
OBSERVATIONS
Experiment 1
A graphical representation of the salient altitudes and the corresponding physiological parameters is depicted in [Figure 3]. Mean SpO2 was 98.97 ± 0.169, mean HR was 97.85 ± 3.38, mean EtCO2 was 42.23 ± 2.353, and mean RR was 20.71 ± 3.59 [Figure 4].
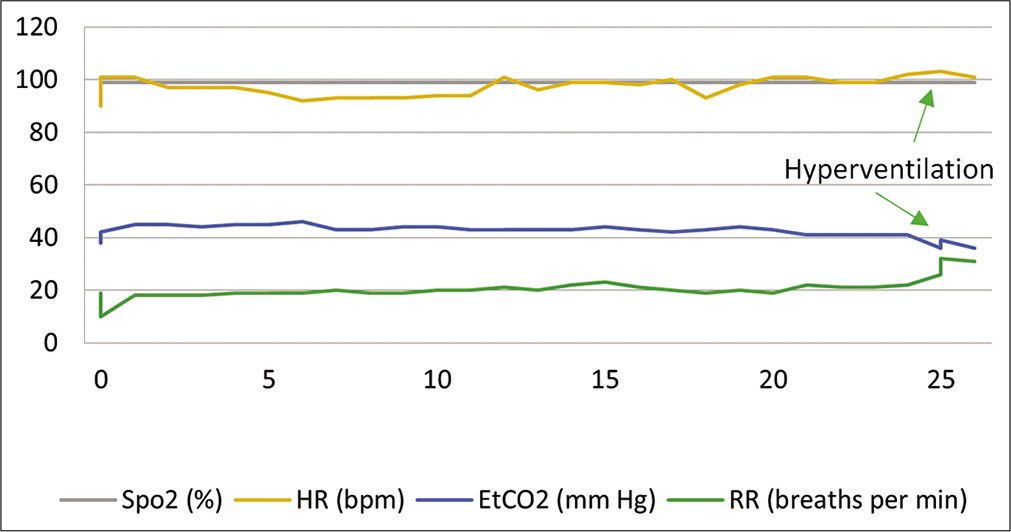
- Physiological parameters on exposure to 25 min at 15,000 ft (Experiment 1).
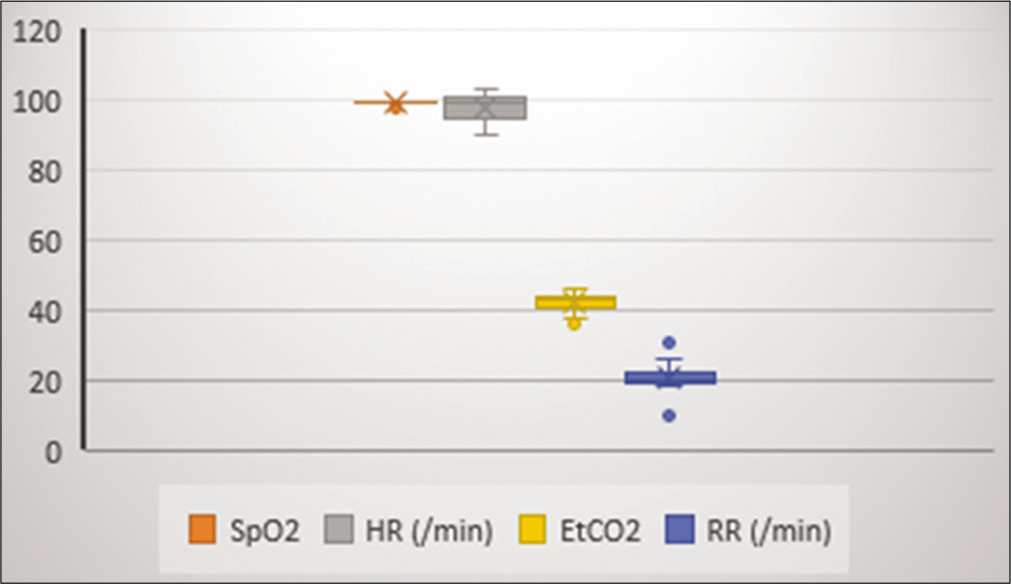
- Mean and SD of physiological parameters (Experiment 1).
Experiment 2
A graphical representation of the salient altitudes and the corresponding physiological parameters is depicted in [Figure 5]. The mean SpO2 was 98.4 ± 0.96, mean HR was 106.7 ± 9.75, mean EtCO2 was 40.17 ± 7.14, and mean RR was 16.8 ± 4.28 [Figure 6].
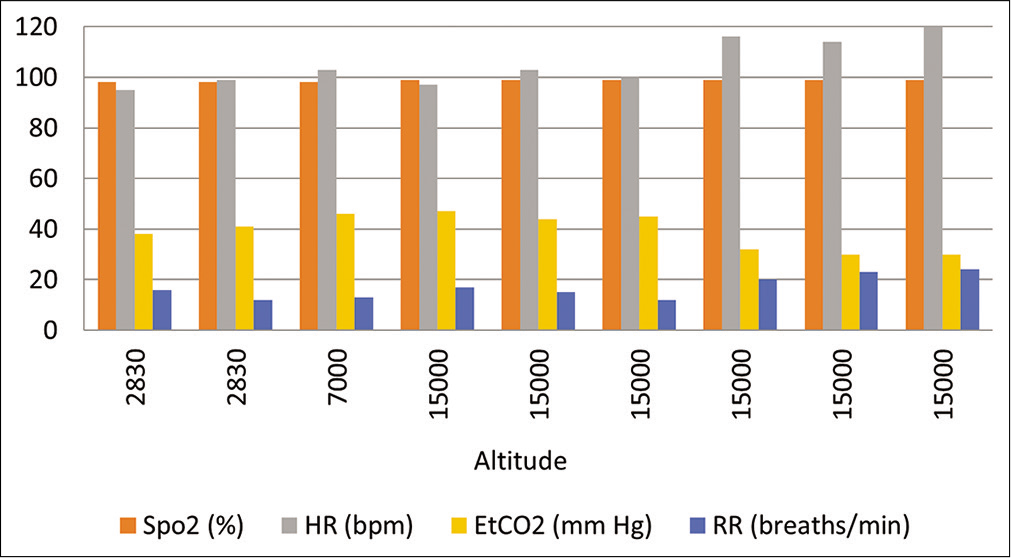
- Altitude versus physiological parameters (Experiment 2) during RD (7000–15,000 ft).
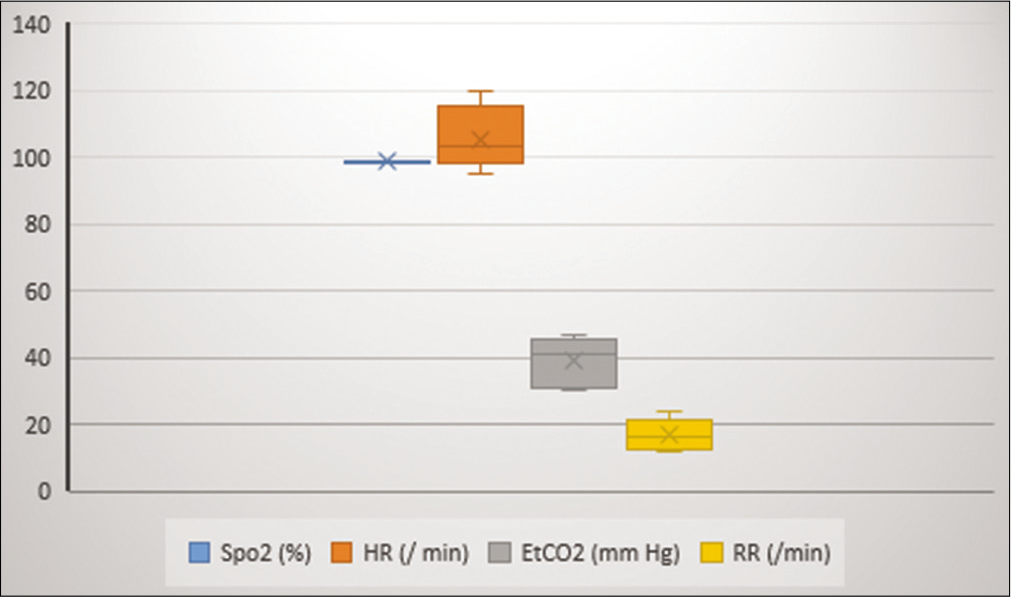
- Mean and SD of physiological parameters (Experiment 2).
Experiment 3
A comparison of the endurance capability of different make oxygen bottles at an altitude of 15,000 ft showed the equivalent time to reach zero bottle pressure which was more than the specified endurance time as given in Tech. Specs [Table 1].
Bottle make | Initial pressure (bar) | Final pressure (bar) | Endurance time (min) | Time specified as per specs (min) |
---|---|---|---|---|
Make 1 | 124 | 0 | 73 | 55 |
Make 2 | 130 | 0 | 73 | 52 |
DISCUSSION
Oxygen equipment in aviation must be capable of meeting the pulmonary ventilation requirements of the user in a variety of situations (hypoxia, smoke in the cockpit, rapid decompression, etc.), both on the ground and during flight.[2] An ideal oxygen system should meet the requirements of an adequate concentration of oxygen, nitrogen, and flow capacity with minimal resistance.[1,2]
The oxygen system of the unpressurised aircraft of Indo-German make was evaluated for retro-fitment as an onboard emergency O2 system in a pressurized aircraft of British vintage. During the ground-based simulator trials, the aeromedical concern of hypoxia, decompression sickness (DCS), endurance, and adequate flow rates was endeavored to be addressed. Three experimental protocols were carried out; the first experiment was checking of the system during exposure to 15,000 ft altitude for 25 min. The second experiment was checking of the system during decompression from 7000 to 15,000 ft. The third experiment checked the endurance of the oxygen system at 15,000 ft.
At an altitude of 15,000 ft, hypoxia is mitigated if the system provides an oxygen percentage of 38%, in the test system which is a non dilution system providing 100% O2. DCS was not a concern as the ceiling limit of the aircraft is capped at 15,000 ft .[3,4]
During the trial to assess the suitability of the test system up to an altitude of 15,000 ft, physiological parameters monitored during the entire duration of the experiment were within normal limits, as shown in [ Figures 3 and 4]. There were no subjective or objective symptoms noted. There was no breathing resistance reported by the subject. Inference can be drawn that the unpressurized aircraft oxygen system is adequate to address the oxygen concentration and flow requirements to counter hypoxia. During hyperventilation by the test subject (RR – 20/min) at the 25th min of the trial which lasted for 1 min, there was no physiological decompensation and EtCO2 values maintained were well below 45 mmHg with no fall in oxygen saturation, as shown in [Figure 3].
The oxygen system being evaluated is a continuous-flow system with a rebreathing reservoir depicted in [Figure 1]. Aircrew seated at rest in an aircraft generally has pulmonary ventilation of 10–15 L/min although with large individual variations.[4] The minimum mass flow required during inspiration should not be less than the flow required to maintain, a mean tracheal oxygen pressure of 149 mmHg when breathing air is 15 L/min BTPS with a maximum tidal volume of 700 ml.[5] This oxygen system has a flow rate of 2.5 L/min of pure 100% oxygen which, according to the FAR regulation [Figure 7], satisfies the requirement of providing a minimum flow rate of 1 L/min at 15,000 ft at normal breathing rates.[5]
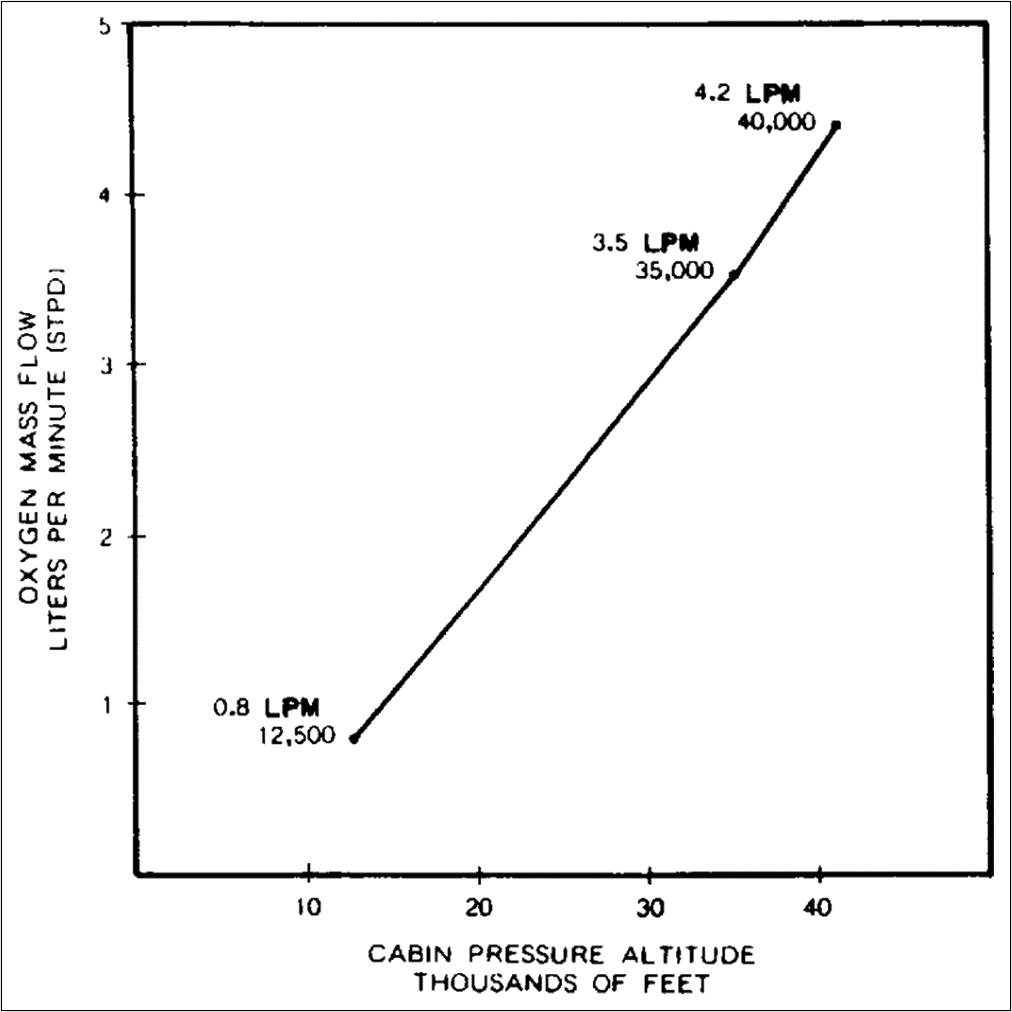
- Cabin altitude versus oxygen flow. Adapted from FAR 23 para 23.1443(a).
In an unpressurised aircraft, the ceiling altitude is capped to prevent exposure of crew and passengers to decompression. However, in pressurized aircraft, a response by aircrew to loss of cabin pressure needs to be evaluated. In a pressurized aircraft, each pilot should use the oxygen continuously when flying for more than 30 min at cabin altitudes above 10,000 ft through 12,000 ft MSL.[6] In the instant study, the second experiment consisted of a simulation of decompression from an altitude of 7000 ft–15,000 ft. Physiological parameters were monitored during the entire duration of the experiment. They were within normal limits with no subjective or objective symptoms noted, as depicted in [Figures 5 and 6]. There was no breathing resistance reported by the subject.
However, in case of a loss in cabin pressure, emergency descent below 10,000 ft is recommended. Ideally, the oxygen system should be able to sustain the aircrew long enough to reach physiologically viable altitudes. The test oxygen system was rated to endure 52 min on a single aircrew and 26 min on two aircrew usage. This was objectively assessed in the EDC by assessing the duration of oxygen consumption and the pressure difference between the beginning and end of the run during the trials. The initial pressure of the unpressurized aircraft oxygen system was 124 bar and 130 bar. Both the systems endured for a total time of 73 min which was substantially more than the specifications.
From the above-simulated trials, it is inferred that unpressurized aircraft oxygen system in a single crew configuration has been found satisfactory for a maximum altitude of 15,000 ft.
CONCLUSION
The ground-based EDC trials revealed that the subject oxygen system in a single crew configuration could provide adequate oxygen to meet the physiological requirement up to an altitude of 15,000 ft and decompression from 7000 to 15,000 ft. In addition, the physiological parameters during voluntary hyperventilation did not reveal any significant abnormalities. In addition, the endurance capability of the systems (in both the configuration) was comparable and was more than that specified in the maintenance manual. Hence, the performance of the system in terms of its functionality and suitability of use in the desired aircraft was considered satisfactory in a single crew configuration. Notwithstanding the above, the system needs to be assessed for its suitability through necessary flight trials in the subject aircraft.
Declaration of patient consent
Institutional Review Board (IRB) permission was obtained for the study.
Financial support and sponsorship
Nil.
Conflicts of interest
Dr. A. V. K. Raju and Dr. Vijay V. Joshi are one of the ISAM Executive Committee Members. Dr. N. K. Tripathy is one of the IJASM Editorial Advisory Board Members.
References
- Hypoxia and Hyperventilation. In: Gradwell DP, ed. Ernsting’s Aviation and Space Medicine (5th ed). Oxford: Butterworth Heinemann; 2016. p. :49-64.
- [Google Scholar]
- The physiological requirements of aircraft oxygen systems In: Gillies JA, ed. A Textbook of Aviation Physiology (1st ed). Oxford: Pergamon Press; 1965. p. :303-42.
- [Google Scholar]
- Hypoxia Induced by Rapid Decompression from 8000 Feet to 40000 Feet: The Influence of Rate of Decompression In: Flying Personnel Research Committee Report No. 1324. London: Ministry of Defence; 1973.
- [Google Scholar]
- Advanced Oxygen Systems for Aircraft In: AGARDograph 286. Neuilly-sur-Seine, France: AGARD/ NATO; 1996.
- [Google Scholar]
- Part 23 Airworthiness Standards: Normal, Utility, Acrobatic, and Commuter Category Airplanes Washington, DC: Federal Aviation Administration; 2011.
- [Google Scholar]
- Part 135 Operating Requirements: Commuter and On Demand Operations and Rules Governing Persons on Board Such Aircraft Washington, DC: Federal Aviation Administration; 2011.
- [Google Scholar]