Translate this page into:
Understanding Magnetic Resonance Imaging
Abstract
Magnetic resonance imaging is a technique that makes use of both magnetic and radiofrequency fields to produce images of the internal structures of the body. The fundamentals of the technique involve placing the patient in a strong magnetic field, stimulation of the atomic nuclei with a radio transmission and the detection of signals from the nuclei as they return to their pre-stimulation state. This technique uses non-ionizing electromagnetic radiation for stimulation and detection of signals, is non-invasive and demonstrates no known untoward biological effects. The available tissue contrast is currently the highest among all imaging modalities. Currently newer techniques in the field of MR imaging like fast spin echo, MR angiography, MR spectroscopy and functional MR imaging provide an unchallenged sensitivity and specificity in the diagnosis of disease process. The most important part of this imaging modality is that it is perhaps still in its growing stage and its horizons appear unlimited.
Keywords
MRI
Aero medical evaluation
Spine
The crux of medical imaging is what makes black things black and white things white. Plain radio graphs are easy to understand; blackness or whiteness is determined by the amount of radiation recorded by the film. In Computed Tomography the image depends solely on electron densities, higher the electron density in a given volume higher is the CT number and brighter is the image.
What makes Magnetic Resonance Imaging (MRI) different is that the same abnormality can appear dark on some MR images and bright on others. The scale of blackness and whiteness in MR imaging is derived not only from inherent tissue properties but also from the specific imaging techniques selected.
Fundamentals of MR instrumentation
The MR machine consists of the following:
A main magnet is used to produce a strong, uniform external magnetic field, powerful enough to induce measurable tissue magnetization. Magnetic field strength is measured in Tesla with most clinical magnets, ranging from 0.2 to 1.5T. This magnet requires large currents thereby producing heat, hence it is necessary to make the entire system extremely cold, which is done by using 'cryogens' like liquid helium or liquid nitrogen, which brings the system temperature to near absolute zero. This process is called super cooling and electro magnets that are super cooled are termed as super conducting magnets [1].
Gradient coils are electromagnetic coils with magnetic field strengths that are only a small fraction of the main magnetic field. They are used to vary the magnetic filed at the center of the main magnet in a deliberate predictable way along each of the perpendicular directions (x, y, z).
Radiofrequency (RF) coils are of two types: transmitter and receiver coils. The transmitter RF coils are used to excite the nucleus within a specific tissue slice by sending precisely timed RF pulse. Receiver coils measure MR signal output from tissue and these vary in size from large whole body to small surface receiver coils. The latter are closely applied to the anatomic region of interest.
Computers for data storing, processing and image display.
Physical principles
MR Imaging depends on a number of physical principles like the magnetic properties of nuclei, the collective behavior of nuclei when excited by radio waves, the relaxation properties of nuclei due to their macromolecular environment, the equipment and the imaging techniques used [2,3].
Charged spinning particles such as protons behave like tiny bar magnets having both north and south poles and produce an external magnetic field. Nuclei with either an odd number of protons or an odd number of neutrons have a net magnetic dipole moment, making the phenomenon of nuclear magnetic resonance possible. The most abundant amongst such nuclei in biological tissue is hydrogen and because of its single unpaired spinning proton, produces the strongest magnetic moment of any element.
In the absence of an external magnetic field, the magnetic dipoles of spinning hydrogen protons are randomly oriented in the body and there is no net magnetization of a tissue. If placed in a very strong externally applied magnetic field Bo, the spinning protons align themselves with or against the field. A slightly greater number of these protons align themselves with the magnetic field and this creates small net magnetization Mo in tissue, which is proportional to the external magnetic field and points along its axis (Fig. 1). It is important to understand that individual protons do not align themselves precisely along the z-axis but preccess around it, analogous to a spinning top [3]. The frequency of precession of the proton is solely dependent on magnetic field strength, the higher the external magnetic field the faster the precess; this relationship between magnetic field and precessional frequency is described by the Larmour equation:
where, w = frequency of precession, r = constant, B = field strength
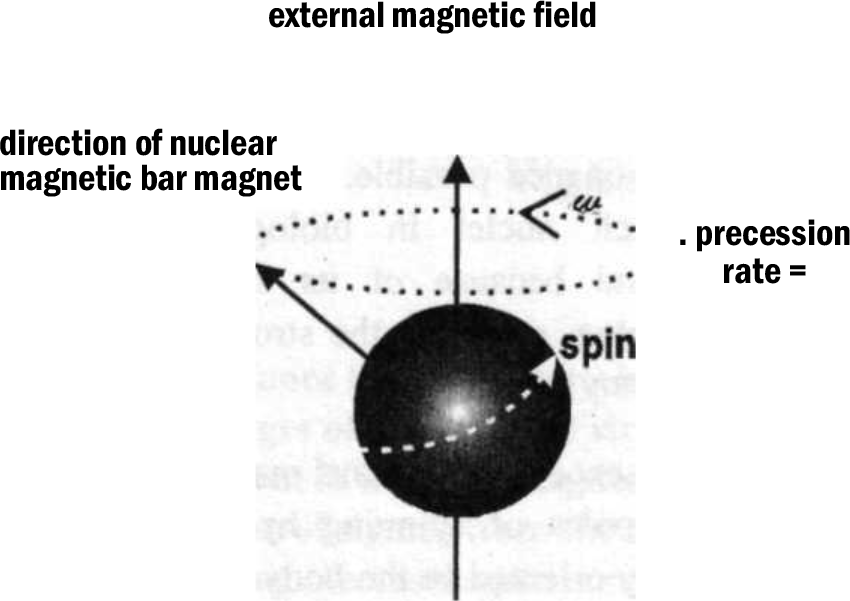
- Fig. 1
For a known magnetic field strength, different nuclei precess at different resonant frequencies and can thus be distinguished from each other. At a field strength of 1 Tesla the resonant frequency of hydrogen nuclei is 42.6 MHz. Therefore at 1 T, 42.6 MHz is the precise frequency of the electromagnetic radiation that must be sent into the patient to excite the hydrogen nuclei and produce the phenomenon of magnetic resonance. It is also the precise frequency to which receiver coils must be tuned to receive outgoing signals from the patient.
How is the signal output measured?
Net intrinsic tissue magnetization Mo is only a tiny fraction of the main external magnetic field and cannot be measured in the longitudinal or z-axis. However this tiny Mo can be measured if it is rotated or tipped away from the longitudinal direction into the perpendicular plane, the transverse or xy plane. This is accomplished by sending a short burst or pulse of electromagnetic radiation oscillating at the Larmour frequency of hydrogen [4]. This burst of radiowaves sent at the Larmour frequency is the R F pulse and is applied for just enough time to tip the net longitudinal magnetization Mo by 90 degrees into the transverse plane, where it can be measured and reconstructed to produce an image.
Immediately after a 90 degree RF pulse has tipped the longitudinal magnetization Mo from its base line state along the longitudinal axis into the transverse plane, longitudinal magnetization is zero and as time progresses, magnetization begins to regrow or recover along the longitudinal direction. This rate of recovery of longitudinal magnetization is described by the relaxation time Tl [4]. Tl is defined as the time required for regrowth or recovery of 63% of the magnetization along the longitudinal direction after the 90-degree RF pulse. Hydrogen protons in different tissue have different Tl recovery rates because of differing macromolecular environment.
After a 90-degree RF pulse, transverse magnetization or Mxy is maximum but with passage of time, precessing dipoles interact with surrounding macromolecules and get out of phase with each other. This is termed dephasing and as dipoles dephase, the net transverse magnetization becomes progressively smaller and this rate of irreversible signal loss is described by a second magnetic relaxation time called T2. T2 relaxation time is the time required for transverse magnetization to decrease to 37% of its original value.
Pulse sequences can be designed to emphasize Tl effect (Tl weighted scan), T2 effects (T2 weighted scan), or balanced (Proton density weighted scan) [3, 4].
Tissues with short Tl will be bright on Tl weighted image (e.g. fat, methaemoglobin Gadolinium), while tissues with long Tl (CSF) will be dark on Tl weighted image. Tissues with short T2 (fat) will be dark on T2 weighted image and tissues with long T2 (Cerebrospinal fluid, cysts, Oedema) will be bright on T2 weigted image.
Pulse sequences : The spin-echo image
The most common basic MR sequence is spin imaging. In this sequence a 90-degree RF pulse is sent into the patient to tip the magnetization into the transverse plane and within a few milliseconds a 180-degree rephasing pulse is applied (Fig. 2).
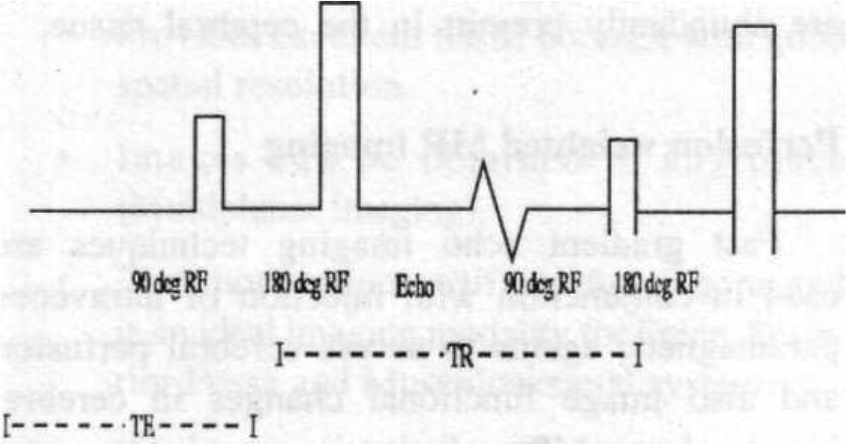
- Fig. 2
The output or signal echoes are measured after a similar time interval following the application of 180-degree rephasing pulse.
This sequence of RF signal —> rephasing pulse —> echo is repeated many times in a single study. The time between repetition of each sequence is the time interval between 90-degree pulses, which is called Time to Repetition (TR).
The time between the middle of 90-degree pulse and the maximum signal output is called the Time to Echo (TE). In general, Tl weighted images provide the best anatomical detail, while T2 weighted images are better for detecting diseases.
Tl Weighted Image - Short TR (<700ms) - Short TE (<20ms)
Tissues with short T like fat, Methhaemoglobin and Gadolinium are bright.
Proton Density Image - Long TR >2000ms - Short TE <30ms
Proton density images have poor tissue discrimination because contrast is primarily due to proton density differences, which vary little from tissue to tissue.
T2 Weighte Image - Long TR (>2000ms) - Long TE (<70-80ms)
Cerebrosphinal fluid and most lesions are bright or hyperintense on a T2 weighted image.
Other pulse sequences:
(a) Inversion Recovery :
The pulse cycle for inversion recovery consists of a 180-degree pulse followed by 90-degree pulse (exactly the reverse of the spin echo) followed by the measurement of signal.
FLAIR (Fluid Attenuated Inversion Recovery) for example is important for separation of lesions from cerebrospinal fluid, since both appear bright on T2 weighted image. With FLAIR the cerebrospinal fluid is made intentionally dark.
(b) Fast scans : (FSE - Fast spin echo, GRE - Gradient recalled echo):
The aim is to reduce the scanning time, which is made possible by -
Using an ultra short Time to Repetition (TR)
Flip angle is less than 90 degree (40-60)
Eliminate the 180 degree reprocessing pulse
Fast scans produce intense signals in arteries and veins and are used for MR Angiography e.g. GRASS (Gradient Recalled Acquisition at Steady State) and FLASH (Fast Low Angle Shot).
Recent developments
MR Angiography
Display of vascular anatomy is generated using sequences where the protons in flowing blood produce a high signal against a background of low or no signal from the stationary tissues. This is accomplished using the “Maximum Intensity Projection” (MIP) algorithm [5]. The computer scans the date by examining the signal intensity in each slice corresponding to each pixel in the plane in which projection is being obtained. Since vascular structures tend to be the brightest tissues on a fast scan image, they will be brightest in the final projection.
MR Spectroscopy
MR Spectroscopy provides a means of obtaining biochemical information non- invasively and improves the specificity of routine MR imaging. Biochemical information is obtained from the MR spectra from a region within imaged brain. The relative or absolute concentration of various biochemical that is obtained produces a type of non-invasive biopsy [6].
This modality relies on information provided by chemical shifts, which are changes in resonance frequency caused due to attenuation in the magnetic field. These changes in the resonance frequencies allow distinctions to be made between the same nuclei in different chemical environment. Data from MR spectroscopy allow for concentrations of various metabolites like choline, creatine, N-acetyl aspartate, lactate, lipids etc to be ascertained. The main application of MR spectroscopy is in disorders of central nervous system. Quantification of the various metabolite peaks indicates the etiological basis of pathology.
The largest peak is due to N-acetyl aspartate and is an indicator of neuronal viability. Decreased levels of Nacetyl aspartate are seen in neoplasms, strokes, multiple sclerosis and dementias. N-acetyl aspartate is elevated only in Canavan's disease. Other dominant peaks are due to Choline and Creatine.
Diffusion Weighted MR Imaging
Using Diffusion Weighted MR Imaging, the extent and location of early ischaemic injury can be assessed within minutes of occlusion, when standard MR shows little or no parenchymal abnormalities. It can also predict which ischaemic tissue is salvageable or irreversibly damaged. In DW Imaging, signals are generated by evaluating the Brownian movement of water molecules, which are abundantly present in the cerebral tissue.
Perfusion weighted MR imaging
Fast gradient echo imaging techniques are used in conjunction with injection of intraveous paramagnetic agents to access cerebral perfusion “and also image functional changes in cerebral blood volume. MR perfusion imaging incorporates recently developed techniques to measure cerebral perfusion non-invasively. This is done by assessment of various haemodynamic measurements such as cerebral blood volume, cerebral blood flow and mean transit time [7].
Clinical application:
Stroke : Identifies viable ischaemic tissue (ischaemic penumbra), in combination with Diffusion Weighted Imaging. The areas of decreased cerebral blood volume, decreased cerebral blood flow and a prolonged mean transit time in the ischaemic region represents both the infarct core and ischaemic tissue at risk, whereas the areas of abnormal diffusion represents the area of irreversible ischaemic infarct core. The mismatch between perfusion and diffusion abnormality is thought to represent the potentially salvageable ischaemic tissue, at risk for infarction. This is important with the availability of recently approved thrombolytic therapy.
Brain Tumors : Cerebral blood volume maps can be used to assess neovascularity in tumors, which is thought to correlate with tumor grade and malignant histology.
Alzheimer's disease : There is a decrease in cerebral blood volume in temporal and parietal lobes in patients with Alzheimer's disease.
Advantages of MRI
Does not use ionising radiation hence is safe with no known biological hazards.
Provides excellent tissue contrast with good spatial resolution.
Images can be obt ai ned in any pl ace (Multiplanar Imaging).
Does not produce artifacts due to bone and is an ideal imaging modality for Spine, Posterior Fossa and Musculoskeletal system.
Does not require use of iodinated contrast media hence there is no risk of anaphylactic reaction.
Contraindications / Precautions
Patients with pacemakers, metallic aneurysm clips and catheters cannot undergo MR imaging.
-
Critically ill patients on life support systems cannot be imaged unless these systems are NCR safe”.
Ilaustrophobia is reported in 2% of patients undergoing MR imaging [3].
Some scanners produce noise that can be overcome by providing “MR safe” hearing protection.
References
- Basic concepts for Nuclear Magnetic Resonance Imaging. Magn Reson Imaging. 1982;1:39-55.
- [Google Scholar]
- Basic Principles and terminology of MRI In: Thomas H, ed. Berquist MRI of the Musculoskeletal system, editor (3rd ed). Lippincott - Raven; 1996. p. :1-37.
- [Google Scholar]
- Introduction to Magnetic Resonance imaging : A Basic Primer (1st ed). Nycorned; 1992.
- [Google Scholar]
- MR Angiography : Basic Principles and Theory. MRI Clinics of North Americal. 1998;2:223-256.
- [Google Scholar]
- In vivo magnetic resonance spectroscopy in humans : A brief review. Am J Physiol Imaging. 1992;3:4-146.
- [Google Scholar]
- MR Perfusion Imaging of the Brain : Techniques and Applications. American Journal of Radiology. 2000;175:207-219.
- [Google Scholar]